Umbilical cord-derived stem cells (MODULATISTTM) show strong immunomodulation capacity compared to adipose tissue-derived or bone marrow-derived mesenchymal stem cells
Abstract
Methods: ModulatistTM was produced from MSCs derived from whole umbilical cord (UC) tissue (which includes Wharton’s jelly and UC), according to GMP compliant procedures. Bone marrow- and adipose tissue-derived MSCs were isolated and proliferated in standard conditions, according to GMP compliant procedures. Immunomodulation mediated by MSCs was assessed by allogenic T cell suppression and cytokine release; role of prostaglandin E2 in the immunomodulation was also evaluated.
Results: The results showed that ModulatistTM exhibited stronger immunomodulation than BMMSC and ADSC in vitro. ModulatistTM strongly suppressed allogeneic T cells proliferation and decreased cytokine production, compared to BMMSCs and ADSCs.
Conclusion: ModulatistTM is a strong immunomodulator and promising MSC product. It may be useful to modulate or treat autoimmune diseases.
Introduction
Mesenchymal stromal cells (MSCs) are multipotent progenitors derived from different sources, including bone marrow (BM) Pham et al., 2014, adipose tissue Van Pham et al., 2014, umbilical cord (UC) blood Sibov et al., 2012, UC Van Pham et al., 2016, Wharton’s jelly Ducret et al., 2016, and other tissues Ducret et al., 2016Pelekanos et al., 2016Sharpe, 2016. Although, bone marrow-derived MSCs (BMMSCs) are a popular source of MSCs for research and clinical applications, there are now alternative sources of MSCs, including adipose tissue-derived stem cells (ADSCs) and umbilical cord-derived mesenchymal stem cells (UCMSCs). ADSCs were first isolated from adipose tissue by Zuk et al., 2001. To date, ADSCs are among the most popular forms of MSCs in clinical research studies. UCMSCs have also made great strides; unlike adipose tissue and BM, UC contains various compartments of stem cells, such as in cord-lining membrane, Wharton’s jelly, cord vein, and blood. Studies have shown that MSCs can be successfully isolated from whole UC or from each compartment Bieback and Netsch, 2016Lim and Phan, 2014Raoufil et al., 2015Watson et al., 2015Zhang et al., 2011. The isolated MSCs successfully differentiate into various functional cells, including adipocytes, osteoblasts, and chondroblasts, and also successfully transdifferentiate into hepatic-like cells, neuron-like cells, and cardiomyocytes. Moreover, MSCs are potent at immunomodulation (i.e. the ability to modulate the immune system).
Immunomodulation by MSCs was discovered more than 10 years ago Bartholomew et al., 2002. One example of MSC-mediated immunomodulation is the capacity of MSCs to inhibit immune cell proliferation. Mechanisms of immunomodulation have been related to cytokine production by MSCs. Due to their ability to modulate immune responses, MSCs have increasingly been studied in the clinic for immunerelated diseases, especially graft-versus-host disease (GVHD). The first stem cells (ProchymalTM) were BMderivedallogenic MSCs, and were approved in 2012 in Canada. Now, several countries are using ProchymalTM to manage GVHD Chen et al., 2014Kurtzberg et al., 2014Prasad et al., 2011. Recently, another form of MSCs was approved in Japan for GVHD Konishi et al., 2016.
MSCs exert immunosuppressive effects in vitro through the regulation of different immune cells. MSCs can suppress lymphocyte activation and proliferation induced by cellular or mitogenic stimuli. In addition to T cells, some studies have shown that B cells, natural killer (NK) cells, and dendritic cells (DCs) are also suppressed by MSCs. Both cell-cell contact and soluble factors from MSCs can participate to modulate immune responses. Essential soluble factors for MSC-mediated immune modulation are hepatic growth factor (HGF), transforming growth factor-b (TGF-b), interleukin (IL)-10, prostaglandin E2 (PGE2), and human leukocyte antigen (HLA) G5.
To meet the increasing desire for “off-the-shelf” stem cell drug production, Modulatist™ cells/products (i.e. Modulatists) were produced from entire UC tissue culture; by this method MSCs could be collected from all compartments of UC tissue. This study aimed to evaluate immunomodulation mediated by Modulatists and to compare it to that of BMMSCs and ADSCs. These results from this study provide useful insight for the role and application of Modulatist™cell products in the clinic.
MATERIALS AND METHODS
Modulatist cell thawing and subculture
Modulatist™ cells (i.e. Modulatists) were produced and cryopreserved by Regenmedlab (Regenmed Co. Ltd., HCM, Vietnam). Modulatists are stem cells derived from whole UC tissue culture under fetal bovine serum (FBS)-free culture medium. Briefly, after primary culture, the stem cells were continuously subcultured up to the 5th passage (to obtain Modulatists), at which point the cells could be cryopreserved. For all studies, cryopreserved Modulatistswere rapidly thawed by immersing the vials into a waterbath at 37°C for 1 min. Then, cells were collected by centrifugation at 1800 rpm/min for 5 min. The pellet was resuspended inFBS-free medium (MSCCult Clinic Completed, Regenmedlab Co. Ltd, HCM, Viet Nam) and cultured in an incubator at 37°C, 5% CO2.
BMMSC isolation from BM
BMMSCs were isolated according to a previously published protocol Pham et al., 2014. Briefly, bone marrow cells were loaded on Ficoll-Hypaque (1.077 g/mL; Sigma-Aldrich, St Louis, MO, USA) and the samples were centrifuged at 3000 rpm for 30 min. Mononuclear cells (MNCs) were collected from the interphase. Collected MNCs were washed twice with PBS and then resuspended in MSCCult Clinic Complete Medium (Regenmedlab Co. Ltd). These primary cells were then subcultured to the 5th passage and used for experiments.
ADSC isolation
ADSCs were isolated following the published protocol Van Pham et al., 2014. Briefly, adipose tissue was digested by collagenase using the ADSC Extraction kit (Geneworld Ltd, HCM, VN). The stromal vascular fractions (SVFs) were then resuspended into MSCCult Clinic Completed Medium (Regenmedlab Co. Ltd). These cells were then sub-cultured to the 5th passage and used for experiments.
Immunophenotyping by flow cytometry
Cell markers were analyzed following a previously published protocol Pham et al., 2014. Briefly, cells were washed twice in PBS containing 1% bovine serum albumin (Sigma-Aldrich, Louis St, MO). The cells were then stained with anti-CD14-FITC, anti- CD34-FITC, anti- CD44-PE, anti-CD45-FITC, anti- CD73-FITC, anti-CD90- PE, anti-CD105-FITC, or anti-HLA-DR-FITC antibody (all antibodies were purchased from BD Biosciences, San Jose, CA, USA). Stained cells were analyzed by FACSCalibur flow cytometer (BD Biosciences). Isotype controls were used in all analyses.
In vitro differentiation
For differentiation into adipogenic cells, MSCs were differentiated as previously described Pham et al., 2014. Briefly, cells from the 5th passage were plated at a density of 1x104 cells/well in 24-well plates. When cells reached 70% confluency, they were cultured for 21 d in DMEM/F12 containing 0.5 mmol/L 3-isobutyl- 1-methyl-xanthine, 1 nmol/L dexamethasone, 0.1 mmol/L indomethacin, and 10% FBS (all purchased from Sigma-Aldrich). Adipogenic differentiation was evaluated by observing lipid droplets in cells, stained with Oil Red, under a microscope.
For differentiation into osteogenic cells, BM- MSCs were plated at a density of 1x104 cells/well in 24- well plates. At 70% confluence, cells were cultured for 21 d in DMEM/F12F12 containing 10% FBS, 10-7mol/L dexamethasone, 50 μmol/L ascorbic acid-2 phosphate, and 10 mmol/L β-glycerol phosphate (all purchased from Sigma-Aldrich). Osteogenic differentiation was confirmed by Alizarin red staining.
Mixed lymphocyte reaction (MLR) and CD38 counting
To evaluate the effects of MSCs on phytohaemagglutinin (PHA)-stimulated allogeneic PBMC proliferation, freshly isolated PBMCs (1 × 105/well) were stimulated with 2.5 μg/ml PHA (Sigma-Aldrich) and added to MSCs cultures, previously seeded and treated with Mitomycin C. Cultures of unstimulated and PHA-stimulated PBMCs seeded without MSCs were used as controls. On day 2, cultures were collected and stained with anti-CD38 monoclonal antibody (BD Bioscience, San Jose) for the final 24 h of culture. The level of proliferation was measured by evaluating the ratio of CD38 positive cells. In some experiments, exogenous PGE2 or PGE2 synthesis inhibitors (indomethacin or NS-398) were added to the culture system. All experiments were performed in triplicate.
ELISA for quantification of human cytokines
Cell-free supernatants were collected and kept frozen at –80°C until assayed for cytokine concentrations by enzyme- linked immunosorbent assay (ELISA). ELISA kits for IL-1β, IFN-γ, IL-2, PGE2 and TNF-α were used following the supplier's instructions (Abcam, Cambrigde, UK).
Statistical analysis
The data were analyzed for statistical significance using GraphPad Prism software. Data were presented as mean ± SEM. When applicable, a Student's unpaired t-test and one-way ANOVA were used to determine significance, p<0.05 was considered to be statistically significant.
Results
Modulatists, BMMSCs, and ADSCs express the MSC phenotype
Modulatists, BMMSCs, and ADSCs all exhibited the MSC phenotype, according to features reported by Dominici et al.(Dominici et al., 2006). The cells were positive for MSC surface markers (CD44, CD73, CD90, and CD105)and negative for non-MSC markers (CD14, CD45 and HLA-DR). There was minimal difference in expression levels of the positive markers in the 3 groups (Modulatists, BMMSCs, and ADSCs) and all groups were able to successfully differentiate into osteoblasts, chondroblasts, and adipocytes ( Figure 1 ).
The differentiated osteoblasts from Modulatists, BMMSCs and ADSCs were stained with alizarin red dye (and were positive), while adipocytes were stained with Oil red. To confirm the differentiation into chondroblasts, Safranin O staining was used; staining was positive. All results were similar to the previous publications Pham et al., 2014Van Pham et al., 2016Van Pham et al., 2014.
Lymphocyte proliferation assay by flow cytometry
ADSCs, BMMSCs, and Modulatists efficiently inhibited T cell proliferation ( Figure 2 ). Compared to with controls (without MSCs), in both 1/40 and ¼ ratios of MSC/T cells, ADSCs, BMMSCs and Modulatist suppressed the T cell proliferation (based on CD38 positive cells). In the control, CD38 positive cells accounted for about 40% in the samples. However, these ratios clearly reduced in condition with ADSC, BMMSCs and Modulatist co-culture (37.66±3.06%, 34±1.73%, 24±5.57% in ratios of 1/40 of MSC/T cells for ADSCs, BMMSCs and Modulatist respectively; 20.67±4.04%, 17.33±2.52%, 12.33±2.52% in ratios of ¼ of MSCs/T cells; for ADSCs, BMMSCs and Modulatist respectively). The results also showed that the T cell proliferation was reduced stronger in Modulatist more than in ADSCs and BMMSCs (p<0.05) ( Figure 2 ).
Analysis of cytokines released by the MSC
The supernatants from PHA-stimulated lymphocytes without MSCs, and PHA-stimulated lymphocytes mixed with MSCs were analyzed for cytokines. The cytokines included IFN-gamma, IL-1 beta, IL-2, and TNF-alpha. The results showed that the supernatant from all groups contained all these cytokines, with the highest amounts found in the PHA-stimulated lymphocytes cultured without MSCs. The cytokine concentrations measured in the PHA-stimulated lymphocyte supernatants were 106.7±20.82 pg/mL for IFN-gamma, 393.7±40.87 pg/mL for TNF-alpha, 40±10 pg/mL for IL-1 beta, and 35±5 pg/mL for IL-2 ( Figure 3 ).
The concentrations of these cytokines became strongly reduced in supernatants of PHA-stimulated lymphocytes that were mixed with MSC cells. The cytokine concentrations were 52±2.65, 26.67±2.52, and 17.33±2.52 pg/mL IFN-gamma, 203.7±25.7, 102±18.08, and 49±5.67pg/mL TNF-alpha, 22±2.65, 9.67±1.53, and 10.33±1.53 pg/mL IL-1 beta, and 15.67±2.08, 6.67±1.53, and 9±1 pg/mL IL-2 in supernatants of PHAstimulated lymphocytes mixed with ADSCs, BMMSCs, and Modulatists, respectively. Overall, these results show that Modulatists produce these cytokines at a significantly lower level than that of ADSCs, and that Modulatists produce IFN-gamma and TNF-alpha at a significantly lower level than do BMMSCs (p<0.05) ( Figure 3 ).
Prostaglandin E2 plays important roles in immunomodulation of Modulatist™
To evaluate the roles of PGE2 in immunomodulation, we neutralized the effects of PGE2 by indomethacin.
The results showed that allogenic T cell proliferation significantly increased in samples with indomethacin. In the groups without indomethacin, compared to control (no MSCs), ADSCs, BMMSCs and Modulatists all significantly suppressed allogenic T cell proliferation ( Figure 4 ). The allogenic T cell proliferation achieved was 45±5%, 30±5%; and 25±5% in ADSC, BMMSC and Modulatist groups, respectively, compared to 100% in control (without MSCs) (p<0.05).
In the indomethacin treatment group, MSCs significantly reduced their suppression of allogenic T cells. The allogenic T proliferation was 75±5%, 60±5%, and 62±7.2% in ADSC, BMMSC and Modulatist groups, respectively, compared to 100% in control (without MSCs). Compared to ‘no indomethacin’ treatment, the percentages were significantly different (45±5% vs 75±5% in ADSCs, 30±5% vs 60±5% in BMMSCs, and 25±5% vs 62±7.2% in Modulatist, respectively (p<0.05). These results show that indomethacin reduced the suppressive potential of MSCs on allogenic T cells ( Figure 4 ).
We measured the PGE2 concentration in the supernatants of Modulatist culture by ELISA. The results showed that under the stimulating conditions (with or without PHA), Modulatists produced and secreted PGE2 into the supernatant. The PGE2 concentration was dependent on the Modulatist cell concentration. In fact, the PGE2 concentration gradually increased according to the increase of Modulatist cell concentrations (103, 5.103, or 2.104 cells) in PHA treatment; PGE2 concentrations were 12±2.65, 230±30, 646.67±50.33, and 793.33±40.42 ng/mL in 0, 103, 5x103, and 2x104 Modulatist cell concentration, respectively. This trend was similar for ‘no PHA’ treatment; PGE2 concentrations were 6±1, 106.67±20.82, 300±50, and 420±26.46 ng/mL in 0, 103, 5x103, and 2x104 Modulatist cell concentration, respectively).
When indomethacin was added to the culture, Modulatists could not produce PGE2; the cytokine was barely detectable in the supernatant (20±10 and 6.66±2.89 ng/mL PGE2, for ‘with PHA’ and ‘without PHA’, respectively) ( Figure 5 ). Notably, PGE2 was produced in the manner that coincided with T cell proliferation. In the PHA treatment group, PHA stimulated allogenic T cell proliferation, and in this group, PGE2 from Modulatists were also produced at a higher amount than that in ‘without PHA’ condition (p<0.05).
Discussion
Stem cell therapy has become a promising therapy for certain degenerative diseases. Although autologous stem cell transplantation holds some advantages, including safety of approach and reduction of virus or disease transmission; it also has disadvantages, namely the uncertainty of stem cell quality. Several studies have shown stem cell quality significantly decreases in older patients, and under certain conditions Beane et al., 2014Nakamura et al., 2016. Therefore, allogeneic stem cell therapy is regarded as a considered as suitable alternative to autologous transplantation. In this study, we evaluated the immunomodulation of stem cell drug that produced from umbilical cord tissue (Modulatist).
Immunomodulation is defined as selective suppression of the immune system (via certain immune cells), while stimulating regulatory T cell (Treg) activity Gea-Banacloche, 2006. The immunomodulatory property of MSCs were discovered more than a decade ago Bartholomew et al., 2002Gotherstrom et al., 2003Le Blanc et al., 2003. Immunomodulation of MSCs can occur via different mechanisms; the most common mechanism occurs via cytokine signals (released by MSCs); the cytokines include IFN-gamma, TNF-alpha, IL-1 beta, and IL-2 De Miguel et al., 2012English, 2013Yagi et al., 2010. These cytokines efficiently inhibit T cell proliferation. The immune modulation of Modulatists in our study was seen to be significantly more potent than that of BMMSCs and ADSCs. Therefore, Modulatists cells can be produced as a “stem cell drug” for immune diseases, especially for autoimmune disease where immune cell activity is overly activated and may need to be dampened.
In the first assay, we identified and confirmed Modulatists as MSCs. Indeed, Modulatists satisfied the criteria of MSCs (as did BMMSCs and ADSCs); they exhibited a fibroblast-like shape when cultured under adherent conditions, they exhibited an MSC marker profile (expression of CD44, CD73, CD90 and CD105), and they were negative for hematopoietic cell markers (e.g. CD14 and CD45). Similar to BMMSCs and ADSCs, Modulatists did not express HLA-DR. Modulatists, BMMSCs, and ADSCs were capable of differentiating into adipocytes, osteoblasts, and chondroblasts. Overall, based on previously published publications Pham et al., 2014Van Pham et al., 2014Vu et al., 2015 and the minimal criteria for MSCs, as suggested by Dominici et al. Dominici et al., 2006, it was confirmed that Modulatistswere indeed MSCs.
The immune modulation of Modulatistwas confirmed by their strong suppression of T cell proliferation. Based on the allogenic T cell proliferation assay, Modulatists showed stronger suppression than BMMSCs or ADSCs. This result was supported by cytokine concentration measurements of mitogenstimulated lymphocytes mixed with Modulatists, BMMSCs, or ADSCs. Although, all 3 groups of MSCs inhibited production of IFN-gamma, TNF-alpha, IL-1 beta and IL-2, the Modulatist cells showed a more significant impact on reduction of cytokine levels.
To address how MSCs suppressed lymphocyte proliferation, we proceeded to evaluate MSCmediated lymphocyte proliferation in two conditions: with or without indomethacin. Indomethacin is a nonsteroidal anti-inflammatory drug that inhibits the production of prostaglandins, including PGE2 Hart and Boardman, 1963Kelly, 1964. In the presence of indomethacin, lymphocyte proliferation was stronger in culture medium without indomethacin. This result shows that indomethacin interfered with MSCmediated suppression of lymphocytes. The results also indicate that the suppression of MSCs is associated with or mediated by PGE2, which has been previously observed for UCMSCs Kim et al., 2015Yu et al., 2014. However, many published publications have also suggested the role of other factors (TGFbeta, HGF, PGE2, NO, and IDO) in immune modulation by MSCs Ma et al., 2014Soleymaninejadian et al., 2012Zhao et al., 2016.
To confirm the role of PGE2 in MSC-mediated immune modulation, we measured the concentration of PGE2 in the supernatants derived from co-culture of Modulatists with lymphocytes in 2 conditions: with or without PHA at varying cell concentrations of Modulatist. PGE2 in the supernatant significantly decreased in indomethacin-supplemented culture. Moreover, PGE2 concentration changed, according to the dose (i.e. cell concentration) of Modulatist. These results demonstrate that PGE2 is the main factor related to suppression of lymphocyte proliferation.
Modulatists are MSCs derived from whole UC culture. Therefore, they contain MSCs from both Wharton’s jelly and UC tissue. This also means that Modulatists are a heterogenous MSC population that exhibits properties of both UCMSCs and Wharton’s jelly-derived MSCs (WJMSCs). As suggested in some previous studies, both UCMSCs and WJMSCs are great prototypes of MSCs with strong immune modulation and low immunogenicity Barcia et al., 2015Li et al., 2014Weiss et al., 2008. Barcia et al. (2015) demonstrated that UCMSCs were less immunogenic and showed higher immunosuppressive activity than BMMSCs Barcia et al., 2015. In fact, Barcia et al. showed that UCMSCs, compared to BMMSCs, show decreased expression of HLA-DRA, HO-1, IGFBP-1, -4 and -6, ILR1, IL6R, and PTGES; however, they show increased expression of CD200, CD273, CD274, IL1B, IL-8, LIF and TGFB2 Barcia et al., 2015. WJMSCs are also a great source of MSCs; several previous publications have shown that WJMSCs exert strong suppression on lymphocyte proliferation and have low immunogenicity Weiss et al., 2008Zhou et al., 2011. In a recent study by Li et al. (2014), the immune modulation of 4 cell populations (BMMSCs, ADSCs, placenta-derived MSCs and WJMSCs) were compared Li et al., 2014. The study concluded that WJMSCs have the strongest immunomodulatory and immunosuppressive potential, as compared to BMMSCs, ADSCs, and placenta-derived MSCs Li et al., 2014. In another study, MSCs from several compartments of UC were isolated Subramanian et al., 2015. The study compared MSCs derived from amnion (AM), subamnion (SA), perivascular (PV), Wharton’s jelly (WJ) and mixed cord (MC) of five UCs. The results suggested that MSCs from WJ are superior than those from PV, SA, AM and MC, in terms of clinical utility and research value. The advantages of WJMSCs included simple isolation procedure, fewer non-SC contaminants, stemness characteristics, high cell production with minimal manipulation, and strong differentiation potential.
Modulatists are MSCs derived from the entire UC, selected using certain specific procedures to isolate and obtain the cells with strongest immunomodulation potential. Our study shows that Modulatists have a strong immunomodulation capacity, greater than BMMSCs and ADSCs. Given their immunomodulatory properties,ModulatistsTMare a promising source of “off-the-shelf” allogenic MSCs for treatment of immune diseases, such as autoimmune diseases.
Conclusion
UC tissue-derived MSCs (Modulatist™) exhibited the standard MSC phenotype; the Modulatist cells expressed the appropriate mesenchymal stem cell markers and profile, and could successfully differentiate into osteoblasts, chondroblasts, and adipocytes. Although Modulatists had a similar phenotype to BMMSCs and ADSCs, they demonstrated a significantly stronger immunomodulation potential and activity. Modulatists efficiently inhibited mitogen-stimulated lymphocyte proliferation and inhibited the production of pro-inflammatory factors (IFN-gamma, TNF-alpha, IL-1 beta and IL-2). The suppression of lymphocyte proliferation was mainly dependent on PGE2. In fact, production of PGE2 by Modulatists was associated with activity level of lymphocytes. These findings suggest that Modulatists are excellent candidates for treatment of immune diseases, such as autoimmune diseases.
Abbreviations
References
-
R.N.
Barcia,
J.M.
Santos,
M.
Filipe,
M.
Teixeira,
J.P.
Martins,
J.
Almeida,
A.
Agua-Doce,
S.C.
Almeida,
A.
Varela,
S.
Pohl.
What Makes Umbilical Cord Tissue- Derived Mesenchymal Stromal Cells Superior Immunomodulators When Compared to Bone Marrow Derived Mesenchymal Stromal Cells?. Stem Cells Int.
2015;
2015
:
583984
.
-
A.
Bartholomew,
C.
Sturgeon,
M.
Siatskas,
K.
Ferrer,
K.
McIntosh,
S.
Patil,
W.
Hardy,
S.
Devine,
D.
Ucker,
R.
Deans.
Mesenchymal stem cells suppress lymphocyte proliferation in vitro and prolong skin graft survival in vivo. Exp Hematol.
2002;
30
:
42-48
.
-
O.S.
Beane,
V.C.
Fonseca,
L.L.
Cooper,
G.
Koren,
E.M.
Darling.
Impact of aging on the regenerative properties of bone marrow-, muscle-, and adipose-derived mesenchymal stem/stromal cells. PLoS One.
2014;
9
:
e115963
.
-
K.
Bieback,
P.
Netsch.
Isolation, Culture, and Characterization of Human Umbilical Cord Blood-Derived Mesenchymal Stromal Cells. Methods Mol Biol.
2016;
1416
:
245-258
.
-
G.L.
Chen,
P.
Paplham,
P.L.
McCarthy.
Remestemcel-L for acute graft-versus-host disease therapy. Expert Opin Biol Ther.
2014;
14
:
261-269
.
-
M.P.
De Miguel,
S.
Fuentes-Julian,
A.
Blazquez-Martinez,
C.Y.
Pascual,
M.A.
Aller,
J.
Arias,
F.
Arnalich-Montiel.
Immunosuppressive properties of mesenchymal stem cells: advances and applications. Curr Mol Med.
2012;
12
:
574-591
.
-
M.
Dominici,
K.
Le Blanc,
I.
Mueller,
I.
Slaper-Cortenbach,
F.
Marini,
D.
Krause,
R.
Deans,
A.
Keating,
D.
Prockop,
E.
Horwitz.
Minimal criteria for defining multipotent mesenchymal stromal cells. The International Society for Cellular Therapy position statement. Cytotherapy.
2006;
8
:
315-317
.
-
M.
Ducret,
H.
Fabre,
O.
Degoult,
G.
Atzeni,
C.
McGuckin,
N.
Forraz,
F.
Mallein-Gerrin,
E.
Perrier-Groult,
J.C.
Fargues.
A standardized procedure to obtain mesenchymal stem/stromal cells from minimally manipulated dental pulp and Wharton’s jelly samples. Bull Group Int Rech Sci Stomatol Odontol.
2016;
53
:
e37
.
-
K.
English.
Mechanisms of mesenchymal stromal cell immunomodulation. mmunol Cell Biol.
2013;
91
:
19-26
.
-
J.C.
Gea-Banacloche.
Immunomodulation. In Principles of Molecular Medicine, M.S. Runge, and C. Patterson, eds.. Totowa, NJ: Humana Press.
2006;
:
893-904
.
-
C.
Gotherstrom,
O.
Ringden,
M.
Westgren,
C.
Tammik,
K.
Le Blanc.
Immunomodulatory effects of human foetal liver-derived mesenchymal stem cells. Bone Marrow Transplant.
2003;
32
:
265-272
.
-
F.D.
Hart,
P.L.
Boardman.
INDOMETHACIN: A NEW NON-STEROID ANTI-INFLAMMATORY AGENT. Br Med J.
1963;
2
:
965-970
.
-
M.
Kelly.
INDOMETHACIN, REMARKABLE ANTIRHEUMATIC DRUG. Med J Aust.
1964;
2
:
541-544
.
-
H.S.
Kim,
J.W.
Yun,
T.H.
Shin,
S.H.
Lee,
B.C.
Lee,
K.R.
Yu,
Y.
Seo,
S.
Lee,
T.W.
Kang,
S.W.
Choi.
Human umbilical cord blood mesenchymal stem cell-derived PGE2 and TGF-beta1 alleviate atopic dermatitis by reducing mast cell degranulation. Stem Cells.
2015;
33
:
1254-1266
.
-
A.
Konishi,
K.
Sakushima,
S.
Isobe,
D.
Sato.
First Approval of Regenerative Medical Products under the PMD Act in Japan. Cell Stem Cell.
2016;
18
:
434-435
.
-
J.
Kurtzberg,
S.
Prockop,
P.
Teira,
H.
Bittencourt,
V.
Lewis,
K.W.
Chan,
B.
Horn,
L.
Yu,
J.A.
Talano,
E.
Nemecek.
Allogeneic human mesenchymal stem cell therapy (remestemcel-L, Prochymal) as a rescue agent for severe refractory acute graft-versus-host disease in pediatric patients. Biol Blood Marrow Transplant.
2014;
20
:
229-235
.
-
K.
Le Blanc,
L.
Tammik,
B.
Sundberg,
S.E.
Haynesworth,
O.
Ringden.
Mesenchymal stem cells inhibit and stimulate mixed lymphocyte cultures and mitogenic responses independently of the major histocompatibility complex. Scand J Immunol.
2003;
57
:
11-20
.
-
X.
Li,
J.
Bai,
X.
Ji,
R.
Li,
Y.
Xuan,
Y.
Wang.
Comprehensive characterization of four different populations of human mesenchymal stem cells as regards their immune properties, proliferation and differentiation. Int J Mol Med.
2014;
34
:
695-704
.
-
I.J.
Lim,
T.T.
Phan.
Epithelial and mesenchymal stem cells from the umbilical cord lining membrane. Cell Transplant.
2014;
23
:
497-503
.
-
S.
Ma,
N.
Xie,
W.
Li,
B.
Yuan,
Y.
Shi,
Y.
Wang.
Immunobiology of mesenchymal stem cells. Cell Death and Differentiation.
2014;
21
:
216-225
.
-
T.
Nakamura,
T.
Hosoyama,
D.
Kawamura,
Y.
Takeuchi,
Y.
Tanaka,
M.
Samura,
K.
Ueno,
A.
Nishimoto,
H.
Kurazumi,
R.
Suzuki.
Influence of aging on the quantity and quality of human cardiac stem cells. Sci Rep.
2016;
6
:
22781
.
-
R.A.
Pelekanos,
V.S.
Sardesai,
K.
Futrega,
W.B.
Lott,
M.
Kuhn,
M.R.
Doran.
Isolation and Expansion of Mesenchymal Stem/Stromal Cells Derived from Human Placenta Tissue. J Vis Exp.
2016
.
-
P.V.
Pham,
N.L.-C.
Phan,
D.M.
Le,
P.T.-B.
Le,
T.D.- X.
Tran,
N.K.
Phan.
Good manufacturing practicecompliant isolation and culture of human bone marrow mesenchymal stem cells. Progress in Stem Cell.
2014;
1
:
10
.
-
V.K.
Prasad,
K.G.
Lucas,
G.I.
Kleiner,
J.A.
Talano,
D.
Jacobsohn,
G.
Broadwater,
R.
Monroy,
J.
Kurtzberg.
Efficacy and safety of ex vivo cultured adult human mesenchymal stem cells (Prochymal) in pediatric patients with severe refractory acute graft-versus-host disease in a compassionate use study. Biol Blood Marrow.
2011;
Transplant17
:
534-541
.
-
A.
Raoufil,
A.
Aminil,
M.
Azadbakht,
F.
Farhadifar,
N.F.
Rahram Nikhn Frrfin Fthi.
Production of hepatocytelike cells from human umbilical vein mesenchymal stem cells. Ital J Anat Embryol.
2015;
120
:
150-161
.
-
P.T.
Sharpe.
Dental mesenchymal stem cells. Development.
2016;
143
:
2273-2280
.
-
T.T.
Sibov,
P.
Severino,
L.C.
Marti,
L.F.
Pavon,
D.M.
Oliveira,
P.R.
Tobo,
A.H.
Campos,
A.T.
Paes,
E. Jr.
Amaro,
F.G.
L.
Mesenchymal stem cells from umbilical cord blood: parameters for isolation, characterization and adipogenic differentiation. Cytotechnology.
2012;
64
:
511-521
.
-
E.
Soleymaninejadian,
K.
Pramanik,
E.
Samadian.
Immunomodulatory properties of mesenchymal stem cells: cytokines and factors. Am J Reprod Immunol.
2012;
67
:
1-8
.
-
A.
Subramanian,
C.Y.
Fong,
A.
Biswas,
A.
Bongso.
Comparative Characterization of Cells from the Various Compartments of the Human Umbilical Cord Shows that the Wharton’s Jelly Compartment Provides the Best Source of Clinically Utilizable Mesenchymal Stem Cells. PLoS One.
2015;
10
:
e0127992
.
-
P.
Van Pham,
N.C.
Truong,
P.T.
Le,
T.D.
Tran,
N.B.
Vu,
K.H.
Bui,
N.K.
Phan.
Isolation and proliferation of umbilical cord tissue derived mesenchymal stem cells for clinical applications. Cell Tissue Bank.
2016;
17
:
289-302
.
-
P.
Van Pham,
B.N.
Vu,
L.-C.N.
Phan,
M.D.
Le,
C.N.
Truong,
H.N.
Truong,
H.-T.K.
Bui,
K.N.
Phan.
Good manufacturing practice-compliant isolation and culture of human adipose derived stem cells. Biomedical Research and Therapy.
2014;
1
:
1-9
.
-
B.N.
Vu,
L.N.
Van Trinh,
T.L.
Phi,
H.T.L.
Vo,
T.T.T.
Dao,
K.N.
Phan,
T.
Van Ta,
P.
Van Pham.
An evaluation of the safety of adipose-derived stem cells. iomedical Research and Therapy.
2015;
2
:
1-7
.
-
N.
Watson,
R.
Divers,
R.
Kedar,
A.
Mehindru,
A.
Mehindru,
M.C.
Borlongan,
C.V.
Borlongan.
Discarded Wharton jelly of the human umbilical cord: a viable source for mesenchymal stromal cells. Cytotherapy.
2015;
17
:
18-24
.
-
M.L.
Weiss,
C.
Anderson,
S.
Medicetty,
K.B.
Seshareddy,
R.J.
Weiss,
I.
VanderWerff,
D.
Troyer,
K.R.
McIntosh.
Immune properties of human umbilical cord Wharton’s jelly-derived cells. Stem Cells.
2008;
26
:
2865-2874
.
-
H.
Yagi,
A.
Soto-Gutierrez,
B.
Parekkadan,
Y.
Kitagawa,
R.G.
Tompkins,
N.
Kobayashi,
M.L.
Yarmush.
Mesenchymal Stem Cells: Mechanisms of Immunomodulation and Homing. Cell transplantation.
2010;
19
:
667-679
.
-
K.R.
Yu,
J.Y.
Lee,
H.S.
Kim,
I.S.
Hong,
S.W.
Choi,
Y.
Seo,
I.
Kang,
J.J.
Kim,
B.C.
Lee,
S.
Lee.
A p38 MAPK-mediated alteration of COX-2/PGE2 regulates immunomodulatory properties in human mesenchymal stem cell aging. PLoS One.
2014;
9
:
e102426
.
-
X.
Zhang,
M.
Hirai,
S.
Cantero,
R.
Ciubotariu,
L.
Dobrila,
A.
Hirsh,
K.
Igura,
H.
Satoh,
I.
Yokomi,
T.
Nishimura.
Isolation and characterization of mesenchymal stem cells from human umbilical cord blood: reevaluation of critical factors for successful isolation and high ability to proliferate and differentiate to chondrocytes as compared to mesenchymal stem cells from bone marrow and adipose tissue. J Cell Biochem.
2011;
112
:
1206-1218
.
-
Q.
Zhao,
H.
Ren,
Z.
Han.
Mesenchymal stem cells: Immunomodulatory capability and clinical potential in immune diseases. Journal of Cellular Immunotherapy.
2016;
2
:
3-20
.
-
C.
Zhou,
B.
Yang,
Y.
Tian,
H.
Jiao,
W.
Zheng,
J.
Wang,
F.
Guan.
Immunomodulatory effect of human umbilical cord Wharton’s jelly-derived mesenchymal stem cells on lymphocytes. Cell Immunol.
2011;
272
:
33-38
.
-
P.A.
Zuk,
M.
Zhu,
H.
Mizuno,
J.
Huang,
J.W.
Futrell,
A.J.
Katz,
P.
Benhaim,
H.P.
Lorenz,
M.H.
Hedrick.
Multilineage cells from human adipose tissue: implications for cellbased therapies. Tissue Eng.
2001;
7
:
211-228
.
Comments
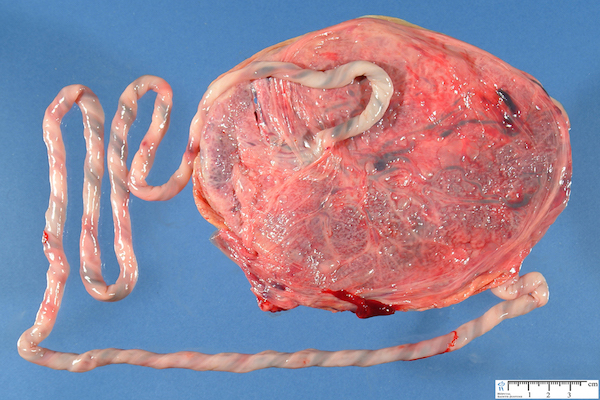
Downloads
Article Details
Volume & Issue : Vol 3 No 06 (2016)
Page No.: 687-696
Published on: 2016-06-26
Citations
Copyrights & License

This work is licensed under a Creative Commons Attribution 4.0 International License.
Search Panel
Pubmed
Google Scholar
Pubmed
Google Scholar
Pubmed
Search for this article in:
Google Scholar
Researchgate
- HTML viewed - 5889 times
- Download PDF downloaded - 1334 times
- View Article downloaded - 16 times