Abstract
Introduction: Cartilage injury is the most common injury among orthopedic diseases. The predominant treatment for this condition is cartilage transplantation. Therefore, production of cartilage for treatment is an important strategy in regenerative medicine of cartilage to provide surgeons with an additional option for treatment of cartilage defects. This study aimed to produce in vitro engineered cartilage tissue by culturing and differentiating umbilical cord derived mesenchymal stem cells on biodegradable Poly(ε-caprolactone) (PCL) scaffold.
Methods: Human umbilical cord derived mesenchymal stem cells (UCMSCs) were isolated and expanded according to previous published protocols. UCMSCs were labeled with CD90 APC‑conjugated monoclonal antibody (CD90-APC) and then seeded onto porous PCL scaffolds. Cell adhesion and proliferation on PCL scaffolds were evaluated based on the strength/signal of APC, MTT assays, and scanning electron microscopy (SEM). The chondrogenic differentiation of UCMSCs on scaffolds was detected by Alcian Blue and Safranin O staining.
Results: The results showed that UCMSCs successfully adhered, proliferated and differentiated into chondroblasts and chondrocytes on PCL scaffolds. The chondrocyte scaffolds were positive for some markers of cartilage, as indicated by Alcian Blue and Safranin O staining.
Conclusion: In conclusion, this study showed successful production of cartilage tissues from UCMSCs on PCL scaffolds.
Introduction
Cartilage lesions are a common problem in the orthopedic practice. In recent years, cartilage lesions (or defects/injury) have been treated by various different strategies, including microfracture Sledge, 2001Steadman et al., 1999, autologous chondrocyte implantation (ACI) Brittberg et al., 1994Podskubka et al., 2006, osteochondral allograft transplantion surgery (OATS) Hangody et al., 1997Okamoto et al., 2007, and stem cell transplantation Bui et al., 2014Nguyen et al., 2017. These treatments have demonstrated some promising results; however, they have also shown some limitations, especially the lack of cartilage tissue for transplantation. Therefore, this study aimed to investigate a potential approach to produce engineered cartilage tissue, using stem cells seeded on scaffolds.
Mesenchymal stem cells (MSCs) have been used to produce cartilage in vitro in previous studies Marmotti et al., 2017. However, there are various kinds of MSCs which can be utilized for differentiation into chondrocytes in vitro. Notably, umbilical cord derived MSCs (UCMSCs) display some useful properties compared to bone marrow derived MSCs or adipose tissue derived MSCs, such as the abundant source of umbilical cord and the strong proliferation and easy isolation of UCMSCS Ding et al., 2015. Moreover, it has previously been reported that UCMSCs can adhere to scaffolds based on architecture and the interactions between cell membranes and scaffold surfaces Guarino et al., 2011.
Poly(ε-caprolactone) (PCL) is a biodegradable polyester Labet and Thielemans, 2009. PCL scaffolds are a bio-scaffold with robust biocompatibility and biodegradation, especially with respect to degradation as it takes about 3 years longer than other scaffolds Woodruff and Hutmacher, 2010. With these characteristics, PCL is thought to be an essential material for cartilage regeneration in tissue engineering applications.
Therefore, this study aimed to produce engineered cartilage in vitro from UCMSCs using PCL scaffolds (and chondrogenesis medium).
Materials - Methods
Poly(ε-caprolactone) scaffold
PCL scaffolds were provided by the Institute of Applied Mechanics and Informatics (Academy of Science and Technology, Vietnam). They have structural pores ranging from 200-300 µm in diameter. PCL scaffolds were sterilized by soaking in alcohol (96%) for one day. Next, the scaffolds were washed in phosphate buffered saline (PBS) and air-dried.
Human umbilical cord derived mesenchymal stem cells
The frozen UCMSCs were produced by our Institute as previously published (Van Pham et al., 2016a Van Pham et al., 2016b). The frozen UCMSCs were thawed at 37°C for 1-2 minutes and then centrifuged at 1000 rpm for 5 minutes to eliminate the cryopreservation medium. The supernatants were then removed and the pellet was re-suspended with 5 ml MSCCult medium containing DMEM/F-12, 10% FBS, 10 ng/ml EGF, 10 ng/ml FGF, 1% antibiotic-antimycotic (all reagents were purchased Thermo Fisher Scientific, Waltham, MA). UCMSCs were cultured in T-25 flasks and incubated at 37°C, 5% CO2.. The medium was changed every 3 days.
MSC phenotype was characterized by flow cytometry using the FACSCalibur machine (BD Biosciences, Franklin Lakes, NJ). UCMSCs were stained with antibodies, including CD14-FITC, CD73-FITC, CD90-FITC, CD105-PE, and HLA-DR-FITC antibodies (purchased from Santa Cruz Biotechnology, Dallas, TX), and CD34-FITC and CD45-FITC antibodies (BD Biosciences, Franklin Lakes, NJ). Cells were stained with appropriate antibodies for 20 min at room temperature. Then, the stained cells were analyzed by BD CellQuest Pro software with a minimum of 10,000 events.
The in vitro ability of UCMSCs to differentiate into adipocytes, osteoblasts and chondroblasts were evaluated by induced media. For adipocyte differentiation, UCMSCs were incubated in DMEM/F12 medium supplemented with 10% FBS, 1% of 100X antibiotic-antimycotic, 10 mM mexamethasone, 2.79 mM indomethacin, 5 mg/ml insulin, and 0.5 M of 1-Methyl-3-isobutylxanthine (IBMX) (Sigma Aldrich, Louis St, MO). To induce differentiation into osteoblasts and chondroblasts, UCMSCs were treated according to the StemPro® Osteogenesis and Chondrogenesis Differentiation Kits (Thermo Fisher Scientific, Waltham, MA), respectively. After 20-30 days of induction, the differentiated cells were assessed by staining with Oil Red O for adipocytes, Alizarin Red S for osteoblasts, and Alcian Blue for chondroblasts (all reagents were purchased from Sigma Aldrich).
Cell adhesion on PCL scaffolds
CD90 APC-labeled UCMSCs (UCMSCs-CD90) were seeded onto PCL scaffolds at a density of about 105-106 cells/ml. UCMSCs-CD90 adhesion on PCL scaffolds was recorded by measurement of fluorescent signal under a fluorescent microscope at excitation wavelength 594 and 633 nm.
Cell proliferation on PCL scaffolds
MTT assay was performed to evaluate the proliferation of UCMSCs on PCL scaffolds. UCMSCs were seeded at a density of 1.2 x 104 cells/PCL scaffold (size 3 x 4 mm). After 3, 7 and 14 days, fresh medium was replaced, then 10µl of MTT solution (0.45 mg/ml) was added. These samples were incubated at 37°C, 5% CO2. After 4 hours, MTT solution was removed and 100 µL DMSO was added (Merck, Germany) to dissolve the formazan crystal. The optical density (OD) values were recorded at 595nm by a DTX-880 system (Beckman Coulter, Brea, CA).
Cell -seeded PCL surface structure change
Cell- seeded PCL surface structure was analyzed by using a scanning electron microscope (SEM) (Hitachi, Tokyo, Japan). UCMSCs (cultured on PCL) was fixed in 4% paraformaldehyde (PFA) (Merck, Kenilworth, NJ) for 30 minutes. This sample was compared to PCL without cells to determine the changing structure of scaffolds in the presence or absence of seeded cells.
Cartilage differentiation of UCMSCs on scaffolds and analysis of aggrecan and glycosaminoglycan expression
The UCMSCs on PCL scaffolds were induced to differentiate into chondrocytes by a commercial induction medium (Chondrogenesis Differentiation Kit), according to the company guidelines. These scaffolds were analyzed after 21 days by Alcian Blue and Safranin O staining (Merck, Kenilworth, NJ). The scaffolds were taken out of medium and fixed in 4% PFA for 30 minutes then rinsed with PBS and soaked in staining solution. For Alcian Blue staining, the scaffolds were stained for 30 minutes then washed with de-staining solution (3C2H5OH: 2CH3COOH), and washed with PBS again to remove all acidic solution. The scaffolds were finally observed under a microscope to determine whether or not aggrecan proteins were expressed. For Safranin O staining, the scaffolds were stained for 10 minutes then washed with 90-95% alcohol. The scaffolds were washed with PBS again and observed under a microscope to determine the presence/expression of glycosaminoglycans (GAGs).
Statistical Analysis
From the data, mean ± SD were calculated. GraphPad Prism Software (San Diego, CA, USA) was used to calculate statistical significance of the results. The difference is considered as significant difference if p<0.05.
Results
Characterization of UCMSCs
After thawing for 3 days, UCMSCs were adherent and had spread on the culture surface. They exhibited fibroblast-like morphology ( Figure 1A ). The abilities of UCMSCs to differentiate in vitro into mesenchymal cells were evaluated by inducing UCMSCs into osteocytes, adipocytes and chondrocytes in 20-30 days. The results showed that in osteogenesis medium, UCMSCs could accumulate calcium, which can bind to Alizarin Red and form red complexes ( Figure 1B ). In adipogenesis medium, UCMSCs formed intracellular lipid droplets that were positive with Oil Red staining assay ( Figure 1C ). In chondrogenesis medium, UCMSCs expressed aggrecans which can link with Alcian Blue to form blue complexes ( Figure 1D ). The cells maintained the surface markers of MSCs- they were positive for CD73, CD90, and CD105, and negative for CD14, CD34, CD45, and HLA-DR ( Figure 1E-M ).
Adhesion of UCMSCs on PCL scaffolds
After 3 days of culture, UCMSCs adhered and expanded on the scaffold surface ( Figure 2B ). UCMSCs adhered and formed cell clusters at the pores of the PCL scaffolds after 7 days ( Figure 2C ). From 21 to 70 days, UCMSCs developed and grew to 80-90% confluence in the pores of the scaffolds ( Figure 2D ). These observations showed that UCMSCs adhered and proliferated well on the PCL scaffold surface. The existence of UCMSCs on the PCL surface was confirmed by SEM capture. As represented in Figure 3, UCMSCs fully covered the surface of PCL ( Figure 3 ).
UCMSC proliferation on PCL scaffolds
After 3 days of culture, UCMSCs began to attach on the surface of PCL scaffolds in the pores. After 7 days, spreading UCMSCs occurred, covering numerous scaffold pores and forming cell clusters. The increase of UCMSCs showed that UCMSCs proliferated from day 3 to day 21 ( Figure 4 ). MTT assay was used as a quantitative method to monitor cell proliferation on PCL scaffolds. From day 3 to day 7, there was a significant increase of OD values. From day 7 to 14, UCMSCs continuously proliferated at a slower rate. Indeed, the OD values at day 14 showed a non-significant increase ( Figure 5 ).
UCMSC differentiation into chondrocytes on PCL scaffolds
After 21 days of differentiation into chondrocytes, UCMSCs changed their shape and showed similar morphology to chondrocytes (rounded and smaller). The cells were strongly positive for Alcian Blue and Safranin O staining ( Figure 6 ), when fixed and stained with these dyes.
Discussion
Engineered cartilage or cartilage engineering is a promising approach to produce adequate cartilage tissues for orthopedic applications, especially for cartilage injury repair. This study aimed to produce engineered cartilage in vitro using UCMSCs and PCL scaffolds. In this study, we showed preliminary success of producing cartilage tissues when culturing UCMSCs on PCL scaffolds, then differentiating them into chondrocytes using suitable inducing medium.
In the first assay, UCMSCs proliferated well and were confirmed to exhibit MSC phenotypes. Indeed, they exhibited the minimal criteria for MSCs, according to the suggested guidelines of the International Society of Cellular Therapy Dominici et al., 2006. More importantly, these cells were confirmed for their chondrocyte differentiation potential in monolayer culture conditions in vitro. They showed successful differentiation into chondrocytes.
Based on this ability, in the next experiment UCMSCs were cultured on PCL scaffolds (the porous structures were made from PCL material). UCMSCs showed they could adhere and proliferate on the surface of these scaffolds. Indeed, this was evident from visualization of UCMSCs on scaffold surface by inverted light microscopy as well as SEM. Data from both microscopes demonstrated that UCMSCs not only strongly adhered but also underwent mitosis and showed robust proliferation on the scaffold structures.
Cellular nuclei staining with DAPI in combination with CD90 marker demonstrated the proliferation of UCMSCs on PCL scaffolds yet also the maintenance of their stemness during the culture. The adherence and proliferation of UCMSCs was also confirmed by the MTT assay. Similarly, Nirmal et al. (2013) and Gauthaman et al. (2011) successfully cultured MSCs in PCL materials and scaffolds Gauthaman et al., 2011Nirmal and Nair, 2013. In a recent study, Xue et al. (2017) also showed that various kinds of MSCs, including UCMSCs, bone marrow MSCs and adipose tissue derived MSCs, can adhere and proliferate on PCL Xue et al., 2017.
After UCMSCs proliferated to about 70-80% confluence on the PCL scaffold surface, they were induced to differentiate into chondrocytes in the same medium conditions that were used to differentiate them in monolayer culture. The results confirmed that after 3 days of induction, UCMSCs on PCL scaffolds started to produce and accumulate GAG proteins that could be detected by Alcian Blue and Safranin O staining. Indeed, Safranin O is a dye which can bind strongly to sulfate groups (SO42-) of GAGs, which has a specific role in chondrogenesis. GAG sulfates appeared in the early phase of chondrocyte formation Demoor et al., 2014. Alcian Blue is a dye that can bind to aggrecans, which appears at the early phase of chondrogenesis. The weakly positive results demonstrated that the induced cells began secreting aggrecans, which is a sign of chondrocyte formation.
From day 7 to day 21, chondrogenesis was more robust- as indicated by stronger positive Alcian Blue and Safranin O staining. Using different scaffolds (alginate gels), Xu et al. (2008) also showed similar results that MSCs could be differentiated into chondrocytes after 6 days of induction Xu et al., 2008. In 2013, Nirmal et al. showed that umbilical cord matrix derived MSCs could proliferate and become differentiated into chondrocytes on polyvinylalcohol-PCL (PVA-PCL) scaffolds Nirmal and Nair, 2013. They also found that the growth factor combination of tumor growth factor beta 3 (TGF-β3) and BMP-2 was more effective for chondrogenesis Nirmal and Nair, 2013. Recently, Xue et al. (2017) showed that UCMSCs, bone marrow derived MSCs and adipose tissue derived MSCs could all be differentiated into osteoblasts on PCL scaffolds Xue et al., 2017.
Conclusion
Cartilage tissue is used frequently in orthopedic surgery, especially for injured cartilage replacement. The limitation of cartilage tissue resources and donors makes it a challenge to repair cartilage injury. Although stem cell therapy can provide some benefits for patients with cartilage injury, due to the impact of cartilage degeneration stem cell therapy can barely regenerate injured cartilage tissue. Thus, cartilage engineering is the new and potentially effective approach to develop cartilage tissue for orthopedic surgery. In this study, we showed some success with producing cartilage tissue by culture and differentiation of umbilical cord derived mesenchymal stem cells on PCL scaffolds using suitable chondrogenesis medium. The engineered cartilage tissues expressed some markers of chondrocytes, as indicated by Alcian Blue and Safranin O staining. Overall, these preliminary results demonstrate that cartilage can be engineered successfully in vitro using UCMSCs on PCL scaffolds. This approach may be a promising method to produce cartilage tissue for orthopedic diseases.
Abbreviations
PACI Autologous Chondrocyte Implantation
CD Cluster of Differentiation
EGF Epidermal Growth Factor
FBS Fetal Bovine Serum
GFG Fibroblast Growth Factor GAGs Glycosaminoglycans
HLA-DR Human Leukocyte Antigen – antigen D Related
IBMX 1-Methyl-3-isobutylxanthine
OATS Osteochondral Allograft Transplantion Surgery
OD Optical Density
PCL Poly(ε-caprolactone)
SD Standard Deviation
SEM Scanning Electron Microscope
UCMSCs Umbilical Cord Derived Mesenchymal Stem Cells
OATS Osteochondral Allograft Transplantion Surgery
Author Contribution
NBV and PVP were responsible for suggesting the idea for this study, creating the experiment design. NVB and PDNN were responsible for analyzing the characterization of MSCs, the adhesion of MSC on PCL, the structure change of PCL and the data, differentiation of MSC into cartilage, writing the result, discussing, preparing the figures, and revising the manuscript. HLTN was responsible for performing the UCMSCs cultures. TTTD was responsible for estimating the cell proliferation. LVG was prepared PCL scaffold. All authors read and approved the manuscript.
References
-
M.
Brittberg,
A.
Lindahl,
A.
Nilsson,
C.
Ohlsson,
O.
Isaksson,
L.
Peterson.
Treatment of deep cartilage defects in the knee with autologous chondrocyte transplantation. The New England Journal of Medicine.
1994;
331(14)
:
889-895
.
View Article PubMed Google Scholar -
K.H.-T.
Bui,
T.D.
Duong,
N.T.
Nguyen,
T.D.
Nguyen,
V.T.
Le,
V.T.
Mai,
N.L.-C.
Phan,
D.M.
Le,
N.K.
Phan,
P.
Van Pham.
Symptomatic knee osteoarthritis treatment using autologous adipose derived stem cells and platelet-rich plasma: a clinical study. Biomedical Research and Therapy 1.
2014;
1
:
02-08
.
View Article Google Scholar -
M.
Demoor,
D.
Ollitrault,
T.
Gomez-Leduc,
M.
Bouyoucef,
M.
Hervieu,
H.
Fabre,
P.
Galera.
Cartilage tissue engineering: Molecular control of chondrocyte differentiation for proper cartilage matrix reconstruction. Biochimica et Biophysica Acta.
2014;
1840(8)
:
2414-2440
.
View Article PubMed Google Scholar -
D.-C.
Ding,
Y.-H.
Chang,
W.-C.
Shyu,
S.-Z.
Lin.
Human umbilical cord mesenchymal stem cells: A new era for stem cell therapy. Cell Transplantation.
2015;
24(3)
:
339-347
.
View Article PubMed Google Scholar -
M.
Dominici,
K.
Le Blanc,
I.
Mueller,
I.
Slaper-Cortenbach,
F.
Marini,
D.
Krause,
E.
Horwitz.
Minimal criteria for defining multipotent mesenchymal stromal cells. The International Society for Cellular Therapy position statement. Cytotherapy.
2006;
8(4)
:
315-317
.
-
K.
Gauthaman,
J. R.
Venugopal,
F. C.
Yee,
A.
Biswas,
S.
Ramakrishna,
A.
Bongso.
Osteogenic differentiation of human Wharton’s jelly stem cells on nanofibrous substrates in vitro. Tissue Engineering. Part A.
2011;
17(1-2)
:
71-81
.
-
V.
Guarino,
M.
Alvarez-Perez,
V.
Cirillo,
L.
Ambrosio.
hMSC interaction with PCL and PCL/gelatin platforms: A comparative study on films and electrospun membranes. Journal of Bioactive and Compatible Polymers.
2011;
26(2)
:
144-160
.
View Article Google Scholar -
L.
Hangody,
G.
Kish,
Z.
Karpati,
I.
Szerb,
I.
Udvarhelyi.
Arthroscopic autogenous osteochondral mosaicplasty for the treatment of femoral condylar articular defects. A preliminary report. Knee surgery, sports traumatology, arthroscopy: official journal of the ESSKA.
1997;
5
:
262-267
.
-
M.
Labet,
W.
Thielemans.
Synthesis of polycaprolactone: A review. Chemical Society Reviews.
2009;
38(12)
:
3484-3504
.
View Article PubMed Google Scholar -
A.
Marmotti,
S.
Mattia,
F.
Castoldi,
A.
Barbero,
L.
Mangiavini,
D. E.
Bonasia,
G. M.
Peretti.
Allogeneic Umbilical Cord-Derived Mesenchymal Stem Cells as a Potential Source for Cartilage and Bone Regeneration: An In Vitro Study. Stem Cells International.
2017;
2017
:
1732094
.
View Article PubMed Google Scholar -
P. D.
Nguyen,
T. D.
Tran,
H. T.
Nguyen,
H. T.
Vu,
P. T.
Le,
N. L.
Phan,
P.
Van Pham.
Comparative Clinical Observation of Arthroscopic Microfracture in the Presence and Absence of a Stromal Vascular Fraction Injection for Osteoarthritis. Stem Cells Translational Medicine.
2017;
6(1)
:
187-195
.
View Article PubMed Google Scholar -
R. S.
Nirmal,
P. D.
Nair.
Significance of soluble growth factors in the chondrogenic response of human umbilical cord matrix stem cells in a porous three dimensional scaffold. European Cells & Materials.
2013;
26
:
234-251
.
View Article PubMed Google Scholar -
Y.
Okamoto,
Y.
Nakagawa,
M.
Maekawa,
M.
Kobayashi,
T.
Nakamura.
Osteochondral grafting for treatment of a massive chondral defect in the knee of a young adult with anterior cruciate ligament deficit. Arthroscopy : the journal of arthroscopic & related surgery : official publication of the Arthroscopy Association of North America and the International Arthroscopy Association.
2007;
23
:
1024.e1021-1024
.
-
A.
Podskubka,
C.
Povýsil,
R.
Kubes,
J.
Sprindrich,
R.
Sedlácek.
[Treatment of deep cartilage defects of the knee with autologous chondrocyte transplantation on a hyaluronic Acid ester scaffolds (Hyalograft C)]. Acta Chirurgiae Orthopaedicae et Traumatologiae Cechoslovaca.
2006;
73(4)
:
251-263
.
PubMed Google Scholar -
S. L.
Sledge.
Microfracture techniques in the treatment of osteochondral injuries. Clinics in Sports Medicine.
2001;
20(2)
:
365-377
.
View Article PubMed Google Scholar -
J. R.
Steadman,
W. G.
Rodkey,
K. K.
Briggs,
J. J.
Rodrigo.
[The microfracture technic in the management of complete cartilage defects in the knee joint]. Der Orthopade.
1999;
28(1)
:
26-32
.
PubMed Google Scholar -
P.
Van Pham,
N. C.
Truong,
P. T.-B.
Le,
T. D.-X.
Tran,
N. B.
Vu,
K. H.-T.
Bui,
N. K.
Phan.
Isolation and proliferation of umbilical cord tissue derived mesenchymal stem cells for clinical applications. Cell and Tissue Banking.
2016a;
17(2)
:
289-302
.
View Article PubMed Google Scholar -
P.
Van Pham,
N. B.
Vu,
N. K.
Phan.
Umbilical cord-derived stem cells (MODULATISTTM) show strong immunomodulation capacity compared to adipose tissue-derived or bone marrow-derived mesenchymal stem cells. Biomedical Research and Therapy.
2016b;
3(6)
:
687-696
.
View Article Google Scholar -
M. A.
Woodruff,
D. W.
Hutmacher.
The return of a forgotten polymer—Polycaprolactone in the 21st century. Progress in Polymer Science.
2010;
35(10)
:
1217-1256
.
View Article Google Scholar -
J.
Xu,
W.
Wang,
M.
Ludeman,
K.
Cheng,
T.
Hayami,
J. C.
Lotz,
S.
Kapila.
Chondrogenic differentiation of human mesenchymal stem cells in three-dimensional alginate gels. Tissue Engineering. Part A.
2008;
14(5)
:
667-680
.
-
R.
Xue,
Y.
Qian,
L.
Li,
G.
Yao,
L.
Yang,
Y.
Sun.
Polycaprolactone nanofiber scaffold enhances the osteogenic differentiation potency of various human tissue-derived mesenchymal stem cells. Stem Cell Research & Therapy.
2017;
8(1)
:
148
.
View Article PubMed Google Scholar
Comments
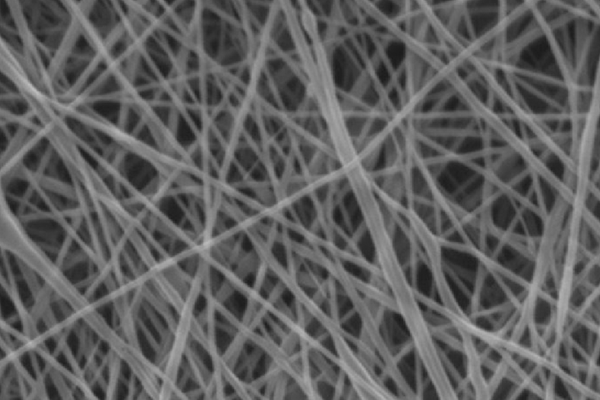
Downloads
Article Details
Volume & Issue : Vol 5 No 02 (2018)
Page No.: 2000-2012
Published on: 2018-02-26
Citations
Copyrights & License

This work is licensed under a Creative Commons Attribution 4.0 International License.
Search Panel
Pubmed
Google Scholar
Pubmed
Google Scholar
Pubmed
Google Scholar
Pubmed
Google Scholar
Pubmed
Google Scholar
Pubmed
Search for this article in:
Google Scholar
Researchgate
- HTML viewed - 8540 times
- Download PDF downloaded - 2431 times
- View Article downloaded - 0 times