Abstract
Background: Diabetes mellitus (DM) is a metabolic disorder that is characterized by hyperglycemia and glucose intolerance, which is associated with impaired insulin secretion and peripheral sensitivity and eventual b-cell dysfunction. This review summarized the major metabolic pathways leading to both microvascular and macrovascular complications in DM, with a view of highlighting the enzymes involved and the possible inhibition of the enzymes facilitating these processes as a measure of diabetic control.
Methods: Data used in writing this review were sourced online from scientific search engines such as Google Scholar, Scopus, EMBASE, PubMed, ResearchGate, Mendeley, Medline, and SpringerLink, using keywords such as 'diabetic complications', 'hyperglycemia-induced diabetic mechanisms', 'diabetic enzymes' and 'diabetic enzyme inhibitors'. A total number of 109 references published online between 1990 and 2020 were generated and cited in this review.
Results: The most scourging and dilapidating effects of DM as well as associated vascular complications are classified into four categories viz.: nephropathy, retinopathy, neuropathy and cardiovascular disease. Hyperglycemia, which is associated with uncontrolled DM, elicits abnormal metabolism such that the enzymes involved in metabolic events leading to diabetic complications are expressed and amplified. The disorders associated with DM are linked to various metabolic pathways facilitated by enzyme activities of the polyol pathway, hexosamine biosynthetic pathway, glucose autoxidation as well as increased synthesis of advanced glycation end-products (AGEs), hexokinase-2 driven glycolytic overload, increased activities of the cyclooxygenase (COX), lipoxygenase (LOX) and pyruvate kinase (PKC) enzymes. The inhibition of the enzymes involved in these pathways could serve to mitigate and arrest diabetic complications.
Conclusion: Thus, suitable inhibitors for enzymes involved in DM metabolic events could serve as panaceas against DM complications, which will add to the growing list of new and more efficacious antidiabetic drugs.
INTRODUCTION
Diabetes mellitus (DM) is a metabolic disorder that is characterized by hyperglycemia and glucose intolerance. It is known to be associated with impaired insulin secretion and peripheral sensitivity, as well as eventual β-cell dysfunction1. DM is one of the oldest diseases worldwide2. The International Diabetes Federation report of 2017 suggested that 451 million adults globally had diabetes in 2017, and 693 million individuals are expected to suffer from DM by 20453. The World Health Organization (WHO) also estimates that more than 19% of the world's total adult population will suffer from DM by the year 20304. DM has been a problem of great concern over the years due to its high incidence and mortality rates, as well as its high management and treatment costs3. DM disorders are more rampant in developing nations, with more than half of the total cases undiagnosed1.
DM is classified into type 1 and type 2. However, DM can also occur during pregnancy- a type known as gestational DM. Other circumstances- such as insulin receptor impairment, pancreatic exocrine disorder, genetic disorders, and endocrinopathies- can provoke DM2. Clinically, type 1 DM presents as hyperglycemia as a result of acute or chronic insulin deficiency in plasma5. In type 2 DM, the β-cells within the islets of Langerhans of the pancreas are hypersensitive to glucose in plasma, thereby eliciting the secretion of higher than normal insulin levels in the systemic circulation. The evidence of hyperinsulinemia is an attempt to counterbalance hyperglycemia, which further deteriorates and impairs β-cell function6, 7. Chronic hyperglycemia is accompanied by high mortality and morbidity due to its concomitant microvascular complications, such as nephropathy, neuropathy and retinopathy, as well as macrovascular complications which include cardiovascular diseases leading to myocardial infarction and stroke2, 8.
Hyperglycemia, which is associated with uncontrolled DM, elicits abnormal metabolism such that the enzymes involved in the metabolic events leading to diabetic complications are expressed and amplified8. Therefore, such enzymes can serve as therapeutic targets for the treatment of DM9. This review summarizes the major metabolic pathways leading to both microvascular and macrovascular complications in DM, and highlights the potential inhibition of the enzymes facilitating these processes as an instrument of diabetic control.
METHODS
Evidence acquisition
Data summarized in this review were sourced online from scientific search engines, including Google Scholar, Scopus, EMBASE, PubMed, ResearchGate, Mendeley, Medline and SpringerLink, using keywords such as 'diabetic complications', 'hyperglycemia-induced diabetic mechanisms', 'diabetic enzymes', and 'diabetic enzyme inhibitors'. A total number of 109 references published online between 1990 and 2020 were evaluated and cited in this review.
RESULTS
Diabetic complications
A significant number of complications accompany DM. However, the most dilapidating effects of DM and its associated vascular complications are classified into four categories, viz.: nephropathy, retinopathy, neuropathy, and cardiovascular disease2.
Nephropathy
Diabetic nephropathy is the main initiator of end-stage renal failure in the Western regions of the world10. Poor glycemic control is a risk factor for the occurrence of diabetic nephropathy11. Clinically, nephropathy is accompanied by an emergence of proteinuria with a concomitant reduction in glomerular filtration rate, leading to fatal uremia if not treated. Kidney disease is also characterized by macrovascular complications, including strokes and heart attacks2. According to Amico and Klein12, a rise in blood pressure is also associated with the onset of nephropathy.
Retinopathy
Diabetic retinopathy is the major cause of blindness in individuals between the ages of 20 – 74 years13, 14 since it initiates an array of lesions in the retina. It is typically characterized by vascular permeability changes, capillary degeneration, capillary microaneurysms, and abnormal production of blood vessels. Color vision deficiency is also another common effect of retinopathy13.
According to Forbes and Cooper2, hyperglycemia induces alteration in the blood-retinal barrier and its vascular permeability at the early stages of diabetic retinopathy. However, the visual disorders that occur at this stage are not noticeable to most sufferers.
Neuropathy
Diabetic neuropathy involves the destruction of the nerves and is one of the most prevalent diabetic complications. More than half of diabetic patients suffer from neuropathy15, 16. Diabetic neuropathy is the main risk factor for wound healing impairment commonly encountered in DM2. According to Obrosova et al.17, advanced diabetic neuropathy, as a result of impairment of the nerve fiber, leads to a total decline in sensory perception. Other complications associated with diabetic neuropathy include erectile dysfunction, cardiovascular dysfunction, paresthesia, hyperalgesia and allodynia2, 17.
Cardiovascular disease
There is a high prevalence of cardiovascular disease among individuals suffering from DM2. Cardiovascular disease is responsible for more than half of the total number of deaths recorded as a result of diabetic complications18. The risk of myocardial infarction among diabetic patients was equivalent to normal human subjects with a previous history of myocardial infarction19. The major disorders associated with cardiovascular disease among diabetic individuals include premature atherosclerosis, accompanied by myocardial infarction, stroke, and cardiac dysfunction2.
Furthermore, cardiovascular disease in type 1 DM occurs sequentially to obstruction in kidney function20, 21. In the same manner, poor glycemic control and kidney disease can provoke cardiovascular disease in type 2 DM22.
Metabolic pathways leading to complications in DM
Certain metabolic processes which are activated by hyperglycemia have been demonstrated to induce the complications associated with DM. These mechanisms, including enzymes and their intermediates, as well as inhibitors of these enzymes, are discussed below.
Protein kinase C (PKC) activation
The PKC family is comprised of more than eleven isoforms of serine-threonine kinases which play a major role in the modulation of endothelial cell permeability, activation of cell proliferation, and vascular growth23. PKC β is the core target in the escalation of diabetic disorders24, 25. The activation of PKC β in diabetic animals and vascular cells is initiated by hyperglycemia24, 25. An increase in blood glucose levels is accompanied by PKC activation in various tissues, including heart, retina, and renal glomeruli, which eventually exacerbates diabetic complications in both humans and animal models (Figure 1)26, 27, 28. High blood glucose levels directly activate the polyol pathway, whereas PKC associated with the polyol pathway is known to induce diabetic complications. Accordingly, the polyol pathway is linked with the generation of oxidative stress, leading to the emergence of diabetic complications as observed clinically (Figure 2)29, 30, 31. Additionally, continuous PKC activation stimulates different growth factors, including transforming growth factor β, platelet-derived growth factor, and vascular endothelial growth factor32, 33.
The study carried out by Thomas et al.,34 on the protein kinase inhibitory activity of crude methanol extract of Bocconia frutescens using hyphae formation inhibition assay against Streptomyces 85E at 20 μg/disk concentration, yielded positive results. The alkaloids- namely chelerythrine and berberine- were reported in this plant extract and were responsible for the protein kinase inhibitory effects of this plant34.
The increased flow of the polyol pathway
The polyol pathway flux consists of two key enzymes: aldose reductase (AR) and sorbitol dehydrogenase (SD). In the polyol pathway, glucose is reduced to sorbitol (alcohol) by AR, followed by the oxidation of sorbitol to fructose by SD. Both the AR- and the SD-catalyzed steps involve using nicotinic acid adenine dinucleotide phosphate (NADPH) (Figure 2)35. The rate of the polyol pathway is dependent on the AR step; AR possesses low glucose affinity (Km > 100 mM) in nondiabetic individuals with normal glucose levels. Indeed, glucose metabolism via the polyol pathway involves the utilization of a very small amount of glucose36.
Under hyperglycemic conditions, AR is activated by rising intracellular glucose levels. The AR reaction eventually leads to the generation of springy polar sorbitol, which permeates the cell membranes, resulting in the distortions of cellular structure and activity, osmotic cell swelling, and reduction in ATPase function23. The oxidation of sorbitol activates PKC to fructose- a process catalyzed by SD- via a rise in NADH/NAD+ ratio37. Oxidative stress is generated in the polyol pathway via redox imbalance38, 39. Thus, the polyol pathway is associated with a vast array of diabetic complications (Figure 2).
Emodin, aurantio-obtusin and chryso-obtusin-2-O-β-D-glucoside, isolated from the ethyl acetate soluble extract of Cassia tora seeds elicited inhibitory effects on AR, with corresponding IC50 of 15.9, 13.6 and 8.8 μmol/L, respectively, against AR in rat lens40. Out of six phytocompounds isolated from Glycyrrhizae radix roots, only isoliquiritigenin gave a strong inhibitory effect on AR as well as blocking the buildup of sorbitol in tissues of diabetic rats41. According to the inhibitory study carried out by Jung et al.,42 twelve phenolic compounds were isolated from Belamcanda chinensis rhizomes, among which tectorigenin and tectoridin gave the most potent and highest inhibitory effect (IC50s = 1.12 and 1.08 μmol/L, respectively). Phenolic compounds blocked the accumulation of sorbitol in streptozotocin-induced diabetic rats within a period of 10 days at a dose of 100 mg/kg42. The AR inhibitory effect of luteolin isolated from Chrysanthemum boreale at IC50 was 0.5 μmol/L43. The phytocompound, 3, 5-dicaffeoylquinic acid (chlorogenic acid derivative), from Ipomoea batatas roots produced a substantial inhibitory effect on AR from rat lens44. Other phytocompounds with AR inhibitory effect include isoaffinetin from Manilkara indica45, rhetsinine from hot water extract of Euodia rutaecarpa46, matteuorienate A and B from Matteuccia orientalis rhizome47, puerariafuran from Pueraria lobata48, and hypolaetin from Sideritis brevibracteata n-butanol extract49.
The increased flow of the hexosamine biosynthetic pathway
The contribution of the hexosamine biosynthetic pathway in the emergence of insulin resistance, as well as diabetic vascular complications, has been reported1, 51. This pathway involves the conversion of fructose-6-phosphate (fruc-6-P) to glucosamine-6-phosphate (glucN-6-P) using glutamine as the amino donor. The conversion of fruc-6-P to glucN-6-P is catalyzed by glutamine: fructose-6-phosphate-amidotransferase (GFAT), which is the rate-limiting enzyme of the hexosamine biosynthetic pathway. GlucN-6-P is instantly channeled towards the synthesis of uridine-5-diphosphate-N-acetylglucosamine (UDP-N-acetylglucosamine). The UDP-N-acetylglucosamine is the precursor for the biosynthesis of the necessary amino sugars required for the generation of glycoproteins, proteoglycans, glycosaminoglycans, and glycolipids51, 52, 53. Extremely high blood sugar levels induce the development of diabetic complications through the elevation of fruc-6-P concentration, which flows into the hexosamine biosynthetic pathway54, 55. However, increased blood glucose concentration induces metabolic pathways that eventually promote the release of cytokines such as TGF-β, ICAM-1, VCAM-1, TNF-α, CTGF and PAI-1, involved in various diabetic complications (Figure 3)56, 57. For instance, transforming growth factor-β1 (TGF-β1) plays a significant role in diabetic nephropathy51. Upon cellular glucose uptake, relatively larger glucose concentrations are catabolized and channeled towards glycogenesis, glycolysis, and pentose phosphate metabolism. Moreover, about 2-3% of glucose molecules are channeled into the hexosamine biosynthetic pathway57, 58.
Nevertheless, the inhibition of the rate-limiting enzyme, GFAT, of the hexosamine biosynthetic pathway blocks the hyperglycemic-induced transcription of the cytokines, thereby preventing the various diabetic complications which might possibly arise from the pathway51, 53, 54, 59.
The photo components present in fenugreek extracts possess an inhibitory effect against the rate-limiting enzyme pathway of the hexosamine biosynthetic pathway60. Diabetic mice fed with fenugreek-containing food showed an inhibited GFAT activity, whereas those given starch diets without fenugreek exhibited an increase in GFAT activity compared with the control group60. The anti-hyperglycemic potency of Euphorbia thymifolia via the inhibition of GFAT has also been reported. The following phytocomponents from E. thymifolia, namely β-amyrin, taraxerol, 1-O-galloyl-β-D-glucose, corilagin, cosmosiin, quercetin-3-galactoside, and quercitrin, all exhibited inhibitory efficacy against GFAT with an absolute binding energy of > 8 kcal/mol61.
Increased synthesis of advanced glycation end-products (AGEs)
AGEs are yellowish-brown fluorescent substances. They are generated via the Maillard reaction. Specifically, they are produced via the non-enzymatic reaction between reducing sugars (e.g., glucose) and the amino group of proteins, leading to synthesis of a Schiff base. The resultant adducts are transiently converted to amadori compounds63. The amadori adducts undergo irreversible dehydration and condensation reactions to yield AGEs63, 64. AGEs are also synthesized from dicarbonyl compounds such as methylglyoxal, 3-deoxyglucosone, and glyoxal, which are outcomes of glucose autoxidation and degradation (Figure 5). Indeed, α-hydroxy aldehydes (including glycolaldehyde and glyceraldehyde) are also precursors for AGE synthesis65, 66. It has been shown that there is increased synthesis and accumulation of AGEs under chronic hyperglycemic conditions, leading to diabetic vascular complications63.
The presence of AGEs induces the expression of AGE receptors. The interaction between AGEs and their receptors elevates cellular generation of oxidative stress, enhances the release of cytokines and growth factors via nuclear factor κB activation, and stimulates adhesion factors, all of which eventually lead to inflammatory response67. This interaction between AGEs and their receptor can furthermore escalate arteriosclerosis progression (Figure 4)64, 68. Aggravations of pathological angiogenesis, reduction in fibrinolytic activity, unstable angina, and/or acute myocardial infarction are other complications associated with an increase in AGE levels69, 70, 71.
Drugs such as atorvastatin, pravastatin, telmisartan, ramipril, rosiglitazone, exendin-4 and aminoguanidine have been reported to elicit modulatory effects on the diabetic complications caused by AGEs72, 73, 74, 75, 76, 77, 78. Atorvastatin, an antioxidant, is known to block the production of AGEs76. Pravastatin is involved in reducing tubular cell destruction in diabetic nephropathy and mitigates cell apoptosis initiated by the AGEs78. The expression of the AGE receptor was blocked by telmisartan in renal mesangial cells, endothelial cells, and liver cells74. Telmisartan also ameliorates the production of oxidative stress, inflammation, and arteriosclerosis associated with AGE expression74, 77. Previously, it has been reported that ramipril elicits similar effects as telmisartan75. In addition, rosiglitazone mitigates the expression of AGE receptor, while exendin-4 inhibits the development of diabetic nephropathy by blocking the interaction between AGEs and their receptors in tubular cells. The inhibition of AGE formation by aminoguanidine has also been reported72, 73.
Glucose autoxidation
Hyperglycemia exacerbates the glucose autoxidation process, which leads to the production of harmful reactive species and ketoaldehyde compounds. Specifically, peroxide (H2O2) and malondialdehyde are generated through this mechanism79.
Hyperglycemia increases the levels of reactive carbonyl species, such as methylglyoxal and glyoxal, as a result of glucose autoxidation54, 80. These reactive carbonyl species can preferentially undergo reactivity with arginine and lysine at relatively high rates (Figure 5), thereby provoking DM pathogenesis. Most of the protein binding sites consist of a large number of arginine residues81, 82. Furthermore, this metabolic process is also linked with the release of AGEs82.
Generally, the aldehyde group of glucose undergoes reactivity with the ε-amino groups of lysine residues and the N-terminal α-amino groups of proteins to produce a Schiff base. Subsequently, it undergoes rearrangement to yield an amadori intermediate. The amadori intermediate is further rearranged for the synthesis of heterogeneous AGEs. The arginine residues of proteins are structurally altered by reactive carbonyl species (Figure 5)82.
Increased expression of cyclooxygenase (COX)
Over the years, COX has been known to exist in the cells of mammals in only two isoforms, namely COX-1 and -283. However, a third isoform known as COX-3 was recently established, although its clinical significance has not been fully confirmed84. COX-1 is the most abundant isoform and occurs in almost all tissues83. COX-2 is released in trace amounts and is induced by growth factors, PKC activation, inflammatory cytokines, oxidative stress, and tumor promoters85, 86, 87.
An increase in COX-1 levels has been linked with DM onset, resulting in heart-related disorders with high mortality rates88. Furthermore, increased expression and activation of COX-2 have been linked with hyperglycemia through glucose autoxidation and AR pathway activation. This pathway is accompanied by secondary NADPH and NAD+ reduction, activation of PKC, stimulation of advanced glycated end-products receptor (RAGE), as well as elevation in reactive oxygen species generation (ROS) (Figure 6)83. According to a study carried out by Guo et al.,89 using type 2 diabetic mice, high COX-2 level was observed in the vascular smooth muscle cells of the mice. An elevation in the abundance of COX-2 in coronary arterioles was also observed and confirmed in diabetic human subjects90, 91. High COX-2 level in the podocytes was also observed and subjected the kidneys to diabetic glomerular injury through a (pro) renin-associated mechanism92.
Inhibition of COX-2 expression was reported to arrest nephropathy in diabetic subjects93, 94, 95. In a related study, the inhibitory action of nimesulide against COX-2 improved endothelial dysfunction in the hind limb vasculature of streptozotocin-induced diabetic rats96. The inhibitory effect of nonsteroidal anti-inflammatory drugs on COX activity has also been extensively reported97, 98.
Activation of lipoxygenase (LOX)
Lipoxygenases (LOX) is a family of enzymes typically characterized by non-heme iron-containing structures and is involved in the catalysis of polyunsaturated fatty acid deoxygenation arachidonic acid to generate hydroperoxyl derivatives, such as hydroperoxy-eicosatetraenoic acids (HPETEs)99.
The 12-LOX (an isoform of lipoxygenase) is activated by hyperglycemia and free fatty acids or pro-inflammatory cytokines. Furthermore, 12-LOX stimulation promotes the release of the pro-inflammatory lipid intermediates, 12 (S)-hydroperoxyeicosatetraenoic acid {12(S)-HPETE} and 12 (S)-Hydroxyeicosatetraenoic acid {12(S)-HETE}. These pro-inflammatory lipid intermediates- in concert with NADPH oxidase (NOX), p38 mitogen-activated protein kinases (p38-MAPK), and c-Jun N-terminal kinase (JNK)- can initiate the activation of inflammatory pathways. Eventually, these signaling pathways cause an elevation in ROS, oxidative stress, and endoplasmic reticulum (ER) stress, which can finally lead to impairment and death of β-cells100. The inhibition of the nuclear factor erythroid 2–related factor 2 (Nrf2) translocation by 12(S)-HETE, which controls the expression of antioxidants, is illustrated in Figure 7100, 101.
ML355 has been reported to be a potent inhibitor of human 12-LOX with an IC50 of 290 nm102. According to Adili et al.,103 ML355 inhibited 12-LOX oxylipin production in vivo in a dose-dependent manner.
Hexokinase-2 driven glycolytic overload
Hexokinase-2 is the rate-limiting enzyme that catalyzes the first step of glycolysis, involving the phosphorylation of glucose to glucose-6-phosphate (G6P)105. Under hyperglycemic conditions, hexokinase-2 initiates an abnormal rise in glycolytic metabolic flux without concurrent transcriptional or other functional regulation. The metabolic flux leads to an unusual increase in the level of glycolytic intermediates. This process is known as a glycolytic overload and is accompanied by several diabetic complications104. The diabetic pathogenic mechanisms associated with this glycolic overload are as follows: G-6-P induces mitochondrial dysfunction, fruc-6-P is channeled to the hexosamine pathway, and dihydroxyacetone phosphate (DHAP) activates PKC. At the same time, AGEs are formed from methylglyoxal (MG) through glyceraldehyde-3-phosphate and DHAP intermediates (Figure 8)104, 106, 107, 108.
However, a possible therapeutic approach towards the prevention of the complications that may arise from glycolytic overload is through the inhibition of G6P buildup and hexokinase-2 displacement from the mitochondria. This can be made possible by channeling G6P towards the pentose phosphate pathway through the stimulation of glucose-6-phosphate dehydrogenase. This process also helps in mitigating carbohydrate response element (ChoRE)-linked expression of hexokinase-2109.
CONCLUSION
The disorders associated with DM are linked to various metabolic pathways, facilitated by enzyme activities of the polyol pathway, hexosamine biosynthetic pathway and glucose autoxidation, as well as being associated with increased synthesis of AGE hexokinase-2 driven glycolytic overload and increased activities of COX, LOX and PKC enzymes. The inhibition of the enzymes involved in these pathways could serve to mitigate and arrest diabetic complications. Thus, suitable inhibitors for enzymes involved in DM metabolic events could serve as panaceas against diabetic complications, and would add to the growing list of new and potentially more effective antidiabetic drugs.
Abbreviations
12-HETE: 12(S)-Hydroxyeicosatetraenoic acid
12-HPETE: 12(S)-hydroperoxyeicosatetraenoic acid
AGEs: Advanced glycation end-products
AR: Aldose reductase
Arg: Arginine
ChoRE: Carbohydrate response element
COX: Cyclooxygenase
DHAP: Dihydroxyacetone phosphate
DM: Diabetes mellitus
eNOS: Endothelial nitric oxide synthase
ER: Endoplasmic reticulum
F-1, 6-bis-P: Fructose-1, 6-bisphosphate
FFAR: Free fatty acid receptor
Fruc-6-P: Fructose-6-phosphate
G6P: Glucose-6-phosphate
GA3P: Glyceraldehyde-3-phosphate
GFAT: Glutamine: fructose-6-phosphate-amidotransferase
Gln: Glutamine
Glu: Glutamate
GLUT1/2: Glucose transporter 1 or 2
IL: Interleukin
JNK: c-Jun N-terminal kinase
LOX: Lipoxygenase
Lys: Lysine
MCP1: Monocyte chemoattractant protein 1
MG: Methylglyoxal
NADPH: Nicotinamide adenine dinucleotide phosphate
NF-kB: Nuclear factor-kappa B
NF-κB: Nuclear factor κB
NOX: Nicotinamide adenine dinucleotide phosphate oxidase
Nrf2: Nuclear factor erythroid 2–related factor 2
OGT: O-linked N-acetylglucosamine (O-GlcNAc) transferase
p38-MAPK: p38 mitogen-activated protein kinases
PGE2: Prostaglandin E2
PKC: Protein kinase C
PLA: Phospholipase A2
RAGE: Advanced glycation end-product receptor
ROS: Reactive oxygen species
SD: sorbitol dehydrogenase
TNF-α: Tumor necrosis factor α
TPI: Triosephosphate isomerase
UDP: Uridine diphosphate
UDP-N-acetylglucosamine: uridine-5-diphosphate-N-acetylglucosamine
Acknowledgments
None.
Authors' contributions
FOO/PCC conceived and designed the scope of the report. FOO/PCC/CMC contributed in writing the paper. FOO/PCC revised and edited the manuscript draft. FOO/PCC/CMC authors were the resource persons who provided all the necessary materials for writing the manuscript. FOO/PCC/CMC approved the manuscript in the present form and gave permission to submit the manuscript for publication.
Funding
None.
Availability of data and materials
Not applicable.
Ethics approval and consent to participate
Not applicable.
Consent for publication
Not applicable.
Competing interests
The authors declare that they have no competing interests.
References
-
Unuofin JO, Lebelo SL. Antioxidant Effects and mechanisms of medicinal plants and their bioactive compounds for the prevention and treatment of type 2 diabetes: An updated review. Oxidative Medicine and Cellular Longevity. 2020; 2020, Article ID 1356893, 36 .
.
View Article PubMed Google Scholar -
Forbes JM, Cooper ME. Mechanisms of diabetic complications. Physiological Review. 2013;93:137-88.
.
View Article PubMed Google Scholar -
International Diabetes Federation (IDF). Diabetes Atlas 2017, 8th edition. 2017
.
-
Danaei G, Finucane MM, Lu Y, Singh GM, Cowan MJ, Paciorek CJ, Lin JK, Farzadfar F, Khang YH, Stevens GA, Rao M, Ali MK, Riley LM, Robinson CA, Ezzati M. National, regional, and global trends in fasting plasma glucose and diabetes prevalence since 1980: Systematic analysis of health examination surveys and epidemiological studies with 370 country-years and 2.7 million participants. Lancet. 2011;378:31-40.
.
View Article Google Scholar -
Mathis D, Vence L, Benoist C. Beta-cell death during progression to diabetes. Nature. 2001;414:792-8.
.
View Article PubMed Google Scholar -
Keenan HA, Sun JK, Levine J, Doria A, Aiello LP, Eisenbarth G, Bonner-Weir S, King GL. Residual insulin production and pancreatic β-cell turnover after 50 years of diabetes: Joslin Medalist Study. Diabetes. 2010;59:2846-53.
.
View Article PubMed Google Scholar -
Cerf ME. Beta-cell dysfunction and insulin resistance. Frontiers in Endocrinology. 2013;4:37.
.
View Article PubMed Google Scholar -
Kitada M, Zhang Z, Mima A, King GL. Molecular mechanisms of diabetic vascular complications. Journal of Diabetes Investigation. 2010;1(3):77-89.
.
View Article Google Scholar -
Alam F, Shafique Z, Amjad ST, BinAsad MHH. Enzymes inhibitors from natural sources with antidiabetic activity: A review. Phytotherapy Research. 2019;33:41-54.
.
View Article PubMed Google Scholar -
Gilbertson DT, Liu J, Xue JL, Louis TA, Solid CA, Ebben JP, Collins AJ. Projecting the number of patients with end-stage renal disease in the United States to the year 2015. Journal of America Society of Nephrology. 2005;16:3736-41.
.
View Article PubMed Google Scholar -
UK Prospective Diabetes Study Group (UKPDS). Intensive blood-glucose control with sulphonylureas or insulin compared with conventional treatment and risk of complications in patients with type 2 diabetes (UKPDS 33). Lancet. 1998;352:837-53.
.
View Article PubMed Google Scholar -
Amico JA, Klein I. Diabetic management in patients with renal failure. Diabetes Care. 1981;4:430-4.
.
View Article PubMed Google Scholar -
Frank RN. Diabetic retinopathy. New England Journal of Medicine. 2004;350:48-58.
.
View Article PubMed Google Scholar -
Hirai FE, Tielsch JM, Klein BE, Klein R. Ten-year change in vision-related quality of life in type 1 diabetes: Wisconsin epidemiologic study of diabetic retinopathy. Ophthalmology. 2011;118:353-8.
.
View Article Google Scholar -
Abbott CA, Malik RA, van Ross ER, Kulkarni J, Boulton AJ. Prevalence and characteristics of painful diabetic neuropathy in a large community-based diabetic population in the UK. Diabetes Care. 2011;34:2220-4.
.
View Article PubMed Google Scholar -
Colloca L, Ludman T, Bouhassira D, Baron R, Dickenson AH, Yarnitsky D, Freeman R, Truini A, Attal N, Finnerup NB, Eccleston C, Kalso E, Bennett DL, Dworkin RH, Raja SN. Neuropathic pain. Nature Reviews Disease Primers. 2017;3:17002.
.
View Article PubMed Google Scholar -
Obrosova IG. Diabetic painful and insensate neuropathy: pathogenesis and potential treatments. Neurotherapy. 2009;6:638-47.
.
View Article Google Scholar -
Laing SP, Swerdlow AJ, Slater SD, Burden AC, Morris A, Waugh NR, Gatling W, Bingley PJ, Patterson CC. Mortality from heart disease in a cohort of 23, 000 patients with insulin-treated diabetes. Diabetology. 2003;46:760-5.
.
View Article PubMed Google Scholar -
Haffner SM, Lehto S, Ronnemaa T, Pyorala K, Laakso M. Mortality from coronary heart disease in subjects with type 2 diabetes and in nondiabetic subjects with and without prior myocardial infarction. New England Journal of Medicine. 1998;339:229-34.
.
View Article PubMed Google Scholar -
Prince CT, Becker DJ, Costacou T, Miller RG, Orchard TJ. Changes in glycemic control and risk of coronary artery disease in type 1 diabetes mellitus: Findings from the Pittsburgh Epidemiology of Diabetes Complications Study (EDC). Diabetologia. 2007;50:2280-8.
.
View Article PubMed Google Scholar -
Groop PH, Thomas MC, Moran JL, Waden J, Thorn LM, Makinen VP, Rosengard-Barlund M, Saraheimo M, Hietala K, Heikkila O, Forsblom C. The presence and severity of chronic kidney disease predicts all-cause mortality in type 1 diabetes. Diabetes. 2009;58:1651-8.
.
View Article PubMed Google Scholar -
Drury PL, Ting R, Zannino D, Ehnholm C, Flack J, Whiting M, Fassett R, Ansquer JC,Dixon P, Davis TM, Pardy C, Colman P, Keech A. Estimated glomerular filtration rate and albuminuria are independent predictors of cardiovascular events and death in type 2 diabetes mellitus: The Fenofibrate Intervention and Event Lowering in Diabetes (FIELD) study. Diabetologia. 2011;54:32-43.
.
View Article PubMed Google Scholar -
Brownlee M. Biochemistry and molecular cell biology of diabetic complications. Nature. 2001;414:813-20.
.
View Article PubMed Google Scholar -
Aiello LP, Cahill MT, Cavallerano JD. Growth factors and protein kinase C inhibitors as novel therapies for the medical management diabetic retinopathy. Eye. 2004;18:117-25.
.
View Article PubMed Google Scholar -
Geraldes P, King GL. Activation of protein kinase C isoforms and its impact on diabetic complications. Circulation Research. 2010;106:1319-31.
.
View Article Google Scholar -
Bennett SP, Griffiths GD, Schor AM, Leese GP, Schor SL. Growth factors in the treatment of diabetic foot ulcers. British Journal of Surgery. 2003;90:133-46.
.
View Article PubMed Google Scholar -
Pathak D, Gupta A, Kamble B, Kuppusamy G, Suresh B. Oral targeting of protein kinase C receptor: promising route for diabetic retinopathy? Current Drug Delivery. 2012;9:405-13.
.
View Article PubMed Google Scholar -
Shi GJ, Shi GR, Zhou J, Zhang W, Gao C, Jiang Y, Zi Z, Zhao H, Yang Y, Yu J (2018) Involvement of growth factors in diabetes mellitus and its complications: A general review. Biomedicine and Pharmacotherapy. 101:510-27.
.
View Article PubMed Google Scholar -
Mathebula SD. Polyol pathway: A possible mechanism of diabetes complications in the eye. African Vision and Eye Health. 74:13. 2015
.
View Article Google Scholar -
Naidu PB, Uddandrao VVS, Naik RR, Pothani S, Munipally PK , Meriga B, Begum MS, Varatharaju C, Pandiyan R, Saravanan G. Effects of S-allylcysteine on biomarkers of the polyol pathway in rats with type 2 diabetes. Canadian Journal of Diabetes. 2016;40:442-8.
.
View Article PubMed Google Scholar -
Sharavana G, Joseph GS, Baskaran V. Lutein attenuates oxidative stress markers and ameliorates glucose homeostasis through polyol pathway in heart and kidney of STZ-induced hyperglycemic rat model. European Journal of Nutrition. 2017;56:2475-85.
.
View Article PubMed Google Scholar -
Koya D, Jirousek MR, Lin YW, Ishii H, Kuboki K, King GL. Characterization of protein kinase C β-isoform activation on the gene expression of transforming growth factor-beta, extracellular matrix components, and prostanoids in the glomeruli of diabetic rats. Journal of Clinical Investigations. 1997;100:115-26.
.
View Article PubMed Google Scholar -
Huang P, Zhang YJ, Huang Y, Zhao JJ, Jiang T, Zhang N. Effect of PKC signaling pathway and aldose reductase on expression of fibronectin induced by transforming growth factor-β1 in human mesangial cells. Chinese Journal of Pathology. 2010;39:405-9.
.
PubMed Google Scholar -
Thomas K, Zhang M, Reinicke J, Parker J, Ohora D, Wall MM, Songsak T, Wongwiwatthananukit S, Chang LC. Protein kinase inhibitory properties of extracts derived from Bocconia frutescens and Gomphocarpus physocarpus. Bulletin of Health Science and Technology. 2017;15:47-58.
.
-
Soriano FG, Virág L, Jagtap P, Szabó É, Mabley JG, Liaudet L, Marton A, Hoyt DG, Murthy KGK, Salzman AL, Southan GJ, Szabó C. Diabetic endothelial dysfunction: the role of poly (ADP-ribose) polymerase activation. Nature Medicine. 2001;7:108-13.
.
View Article PubMed Google Scholar -
Prnova MS, Ballekova J, Gajdosikova A, Gajdosik A, Stefek M. A novel carboxymethylated mercaptotriazinoindole inhibitor of aldose reductase interferes with the polyol pathway in streptozotocin-induced diabetic rats. Physiology Research. 2015;64:587-91.
.
View Article PubMed Google Scholar -
Boesten DMPHJ, Ungern-Sternberg SNIV, Hartog GJMD, Bast A. Protective pleiotropic effect of flavonoids on NAD+ levels in endothelial cells exposed to high glucose. Oxidative Medicine and Cellular Longevity. 2015;2015, Article ID 894597, 7 pages.
.
View Article PubMed Google Scholar -
Shakeel M. Recent advances in understanding the role of oxidative stress in diabetic neuropathy. Diabetes and Metabolic Syndrome: Clinical Research and Review. 2015;9:373-8.
.
View Article PubMed Google Scholar -
Li F, Zhao YB, Wang DK, Zou X, Fang K, Wang KF. Berberine relieves insulin resistance via the cholinergic anti-inflammatory pathway in HepG2 cells. Jouranl of Huazhong University of Science and Technology-Medical Science. 2016;36:64-69.
.
View Article PubMed Google Scholar -
Jang DS, Yoo NH, Kim JM, Lee YM, Yoo JL, Kim YS, Kim JS. An ellagic acid rhamnoside from the roots of Potentilla discolor with protein glycation and rat lens aldose reductase inhibitory activity. Natural Product Science. 2007;13:160-3.
.
-
Aida K, Tawata M, Shindo H, Onaya T, Sasaki H, Yamaguchi T, Chin M, Mitsuhashi H. Isoliquiritigenin: A new aldose reductase inhibitor from Glycyrrhizae radix. Planta Medicine. 1990;56:254-8.
.
View Article PubMed Google Scholar -
Jung SH, Lee YS, Lee S, Lim SS, Kim YS, Shin KH. Isoflavonoids from the rhizomes of Belamcanda chinensis and their effects on aldose reductase and sorbitol accumulation in streptozotocin induced diabetic rat tissues. Archives of Pharmacal Research. 2002;25:306-12.
.
View Article PubMed Google Scholar -
Shin KH, Kang SS, Seo EA, Shin SW. Isolation of aldose reductase inhibitors from the flowers of Chrysanthemum boreale. Archives of Pharmacal Research. 1995;18:65.
.
View Article Google Scholar -
Fuente JA, Manzanaro S. Aldose reductase inhibitors from natural sources. Natural Product Reports. 2003;20:243-51.
.
View Article PubMed Google Scholar -
Haraguchi H, Hayashi R, Ishizu T, Yagi A. A flavone from Manilkara indica as a specific inhibitor against aldose reductase in vitro. Planta Medicine. 2003;69:853-5.
.
View Article PubMed Google Scholar -
Kato A, Yasuko H, Goto H, Hollinshead J, Nash RJ, Adachi I. Inhibitory effect of rhetsinine isolated from Evodia rutaecarpa on aldose reductase activity. Phytomedicine. 2009;16:258-61.
.
View Article PubMed Google Scholar -
Kadota S, Basnet P, Hase K, Namba T. Matteuorienate A and B, two new and potent aldose reductase inhibitors from Matteuccia orientalis. Chemical and Pharmaceutical Bulletin. 1994;42:1712-4.
.
View Article PubMed Google Scholar -
Kim NH, Kim YS, Lee YM, Jang DS, Kim JS. Inhibition of aldose reductase and xylose-induced lens opacity by puerariafuran from the roots of Pueraria lobata. Biological and Pharmaceutical Bulletin. 2010;33:1605-9.
.
View Article PubMed Google Scholar -
Guvenc A, Okada Y, Akkol EK, Duman H, Okuyama T, Calis I. Investigations of anti-inflammatory, anti-nociceptive, antioxidant and aldose reductase inhibitory activities of phenolic compounds from Sideritis brevibracteata. Food Chemistry. 2010;118:686-92.
.
View Article Google Scholar -
Digiacomo M, Sartini S, Nesi G, Sestito S, Coviello V, LaMotta C, Rapposelli S. Synthesis and functional evaluation of novel aldose reductase inhibitors bearing a spirobenzopyran scaffold. Open Journal of Medical Chemistry. 2017;11:9-23.
.
View Article PubMed Google Scholar -
Schleicher ED, Weigert C. Role of the hexosamine biosynthetic pathway in diabetic nephropathy. Kidney International. 2000;58:S13-8.
.
View Article Google Scholar -
Marshall S, Bacote V, Traxinger RR. Discovery of a metabolic pathway mediating glucose-induced desensitization of the glucose transport system: Role of hexosamine biosynthesis in the induction of insulin resistance. Journal of Biological Chemistry. 1991;266:4706-12.
.
PubMed Google Scholar -
Kolm-Litty V, Sauer U, Nerlich A, Lehmann R, Schleicher ED. High glucose-induced transforming growth factor β-1 production is mediated by the hexosamine pathway in porcine glomerular mesangial cells. Journal of Clinical Investigation. 1998;101:160-9.
.
View Article PubMed Google Scholar -
Giacco F, Brownlee M. Oxidative stress and diabetic complications. Circulation Research. 2010;107:1058-70.
.
View Article Google Scholar -
Beriault D, Shi Y, Werstuck G. Investigating the role of the hexosamine biosynthesis pathway in diabetic atherosclerosis. Canadian Journal of Cardiology. 2013;29:S254.
.
View Article Google Scholar -
Marsh SA, DellItalia LJ, Chatham JC. Activation of the hexosamine biosynthesis pathway and protein O-GlcNA-acylation modulate hypertrophic and cell signaling pathways in cardiomyocytes from diabetic mice. Amino Acids. 2011;40:819-28.
.
View Article PubMed Google Scholar -
Taparra K, Tran PT, Zachara NE. Hijacking the hexosamine biosynthetic pathway to promote EMT-mediated neoplastic phenotypes. Frontiers in Oncology. 2016;6.
.
View Article PubMed Google Scholar -
Chiaradonna F, Ricciardiello F, Palorini R. The nutrient-sensing hexosamine biosynthetic pathway as the hub of cancer metabolic rewiring. Cells. 2018;7:53.
.
View Article PubMed Google Scholar -
Pang Y, Bounelis P, Chatham JC, Marchase RB. Hexosamine pathway is responsible for inhibition by diabetes of phenylephrine-induced inotropy. Diabetes. 2004;53:1074-81.
.
View Article PubMed Google Scholar -
Shetty AK, Salimath PV. Reno-protective effects of fenugreek (Trigonella foenumgreacum) during experimental diabetes. e-SPEN Journal. 2009;4:e137-42.
.
View Article Google Scholar -
Nguyen VTH, Tran N, Nguyen D, Le L. An in silico study on antidiabetic activity of bioactive compounds in Euphorbia thymifolia Linn. SpringerPlus. 2016;5:1359.
.
View Article PubMed Google Scholar -
Vyas B, Silakari O, Singh BM, Singh B. Glutamine: fructose-6-phosphate amidotransferase (GFAT): Homology modelling and designing of new inhibitors using pharmacophore and docking based hierarchical virtual screening protocol. SAR and QSAR in Environmental Research. 2013;24:733-52.
.
View Article Google Scholar -
Yamagishi S, Imaizumi T. Diabetic vascular complications: Pathophysiology, biochemical basis and potential therapeutic strategy. Current Pharmaceutical Design. 2005;11:2279-99.
.
View Article PubMed Google Scholar -
Rhee SY, Kim YS. The Role of advanced glycation end products in diabetic vascular complications. Diabetes and Metabolism Journal. 2018;42:188-95.
.
View Article PubMed Google Scholar -
Lo TW, Westwood ME, McLellan AC, Selwood T, Thornalley PJ. Binding and modification of proteins by methylglyoxal under physiological conditions. A kinetic and mechanistic study with N alpha-acetylarginine, N alpha-acetylcysteine, and N alpha-acetyllysine, and bovine serum albumin. Journal of Biological Chemistry. 1994;269:32299-305.
.
View Article PubMed Google Scholar -
Ahmed N, Thornalley PJ, Dawczynski J, Franke S, Strobel J, Stein G, Haik GM. Methylglyoxal-derived hydroimidazolone advanced glycation end-products of human lens proteins. Investigative Ophthalmology and Visual Science. 2003;44:5287-92.
.
View Article PubMed Google Scholar -
Naka Y, Bucciarelli LG, Wendt T, Lee LK, Rong LL, Ramasamy R, Yan SF, Schmidt AM. RAGE axis: Animal models and novel insights into the vascular complications of diabetes. Arteriosclerosis Thrombosis and Vascular Biology. 2004;24:1342-9.
.
View Article PubMed Google Scholar -
Xu B, Ji Y, Yao K, Cao YX, Ferro A. Inhibition of human endothelial cell nitric oxide synthesis by advanced glycation end-products but not glucose: relevance to diabetes. Clinical Science (London). 2005;109:439-446.
.
View Article PubMed Google Scholar -
Yamagishi S, Adachi H, Takeuchi M, Enomoto M, Furuki K, Matsui T, Nakamura K, Imaizumi T. Serum level of advanced glycation end-products (AGEs) is an independent determinant of plasminogen activator inhibitor-1 (PAI-1) in nondiabetic general population. Hormone and Metabolic Research. 2007;39:845-8.
.
View Article PubMed Google Scholar -
Singh VP, Bali A, Singh N, Jaggi AS. Advanced glycation end products and diabetic complications. Korean Journal of Physiology and Pharmacology. 2014;18:1-14.
.
View Article PubMed Google Scholar -
Yamagishi S. Role of advanced glycation end products (AGEs) and receptor for AGEs (RAGE) in vascular damage in diabetes. Experimental Gerontology. 2011;46:217-24.
.
View Article PubMed Google Scholar -
Thornalley PJ, Yurek-George A, Argirov OK. Kinetics and mechanism of the reaction of aminoguanidine with the alpha-oxoaldehydes glyoxal, methylglyoxal, and 3-deoxyglucosone under physiological conditions. Biochemical Pharmacology. 2000;60:55-65.
.
View Article Google Scholar -
Forbes JM, Yee LT, Thallas V, Lassila M, Candido R, Jandeleit-Dahm KA, Thomas MC, Burns WC, Deemer EK, Thorpe SR, Cooper ME, Allen TJ. Advanced glycation end product interventions reduce diabetes-accelerated atherosclerosis. Diabetes. 2004;53:1813-23.
.
View Article Google Scholar -
Yamagishi S, Nakamura K, Matsui T. Potential utility of telmisartan, an angiotensin II type 1 receptor blocker with peroxisome proliferator-activated receptor-gamma (PPAR-gamma)-modulating activity for the treatment of cardiometabolic disorders. Current Molecular Medicine. 2007a;7:463-69.
.
View Article Google Scholar -
Coughlan MT, Thallas-Bonke V, Pete J, Long DM, Gasser A, Tong DC, Arnstein M, Thorpe SR, Cooper ME, Forbes JM. Combination therapy with the advanced glycation end product cross-links breaker, alagebrium, and angiotensin converting enzyme inhibitors in diabetes: synergy or redundancy? Endocrinology. 2007;148:886-95.
.
View Article Google Scholar -
Yamagishi S. Possible involvement of advanced glycation end products in carry-over benefits of atorvastatin in ASCOT-BPLA. European Heart Journal. 2008;29:1922.
.
View Article Google Scholar -
Yamagishi S, Nakamura K, Matsui T. Regulation of advanced glycation end product (AGE)-receptor (RAGE) system by PPAR-gamma agonists and its implication in cardiovascular disease. Pharmacology Research. 2009;60:174-8.
.
View Article PubMed Google Scholar -
Ishibashi Y, Yamagishi S, Matsui T, Ohta K, Tanoue R, Takeuchi M, Ueda S, Nakamura K, Okuda S. Pravastatin inhibits advanced glycation end products (AGEs)-induced proximal tubular cell apoptosis and injury by reducing receptor for AGEs (RAGE) level. Metabolism. 2012;61:1067-72.
.
View Article Google Scholar -
Yaribeygi H, Atkin SL, Sahebkar A. A review of the molecular mechanisms of hyperglycemia-induced free radical generation leading to oxidative stress. Journal of Cell Physiology. 2019;234:1300-1312.
.
View Article PubMed Google Scholar -
Thornalley PJ, Langborg A, Minhas HS. Formation of glyoxal, methylglyoxal and 3-deoxyglucosone in the glycation of proteins by glucose. Biochemistry Journal. 1999;344:109-16.
.
View Article Google Scholar -
Rabbani N, Thornalley PJ. Methylglyoxal, glyoxalase 1 and the dicarbonyl proteome. Amino Acids. 2010;42:1133-42.
.
View Article PubMed Google Scholar -
Chetyrkin S, Mathis M, Pedchenko V, Sanchez OA, McDonald WH, Hachey DL, Madu H, Stec D, Hudson B, Voziyan P. Glucose autoxidation induces functional damage to proteins via modification of critical arginine residues. Biochemistry. 2011;50:6102-12.
.
View Article PubMed Google Scholar -
Kellogg AP, Cheng, HT, Pop-Busui R. Cyclooxygenase-2 pathway as a potential therapeutic target in diabetic peripheral neuropathy. Current Drug Targets. 2008;9:68-76.
.
View Article PubMed Google Scholar -
Kis B, Snipes JA, Busija DW. Acetaminophen and the cyclooxygenase-3 puzzle: Sorting out facts, fictions, and uncertainties. Journal of Pharmacology and Experimental Therapeutics. 2005;315:1-7.
.
View Article PubMed Google Scholar -
Cosentino F, Eto M, De Paolis P, van der Loo B, Bachschmid M, Ullrich V, Kouroedov A, Delli Gatti C, Joch H, Volpe M, Luscher TF. High glucose causes upregulation of cyclooxygenase-2 and alters prostanoid profile in human endothelial cells: Role of protein kinase C and reactive oxygen species. Circulation. 2003;107:1017-23.
.
View Article Google Scholar -
Harris RE. Cyclooxygenase-2 (cox-2) and the inflammogenesis of cancer. Subcell Biochemistry. 2007;42:93-126.
.
View Article PubMed Google Scholar -
Xu K, Shu HK. EGFR activation results in enhanced cyclooxygenase-2 expression through p38 mitogen-activated protein kinase-dependent activation of the Sp1/Sp3 transcription factors in human gliomas. Cancer Research. 2007;67:6121-9.
.
View Article PubMed Google Scholar -
Verma S, Chandra H, Banerjee M. Cyclooxygenase 1 (COX1) expression in type 2 diabetes mellitus: a preliminary study from North India. Egypt Journal of Medicine and Human Genetics. 2016;17:41-5.
.
View Article Google Scholar -
Guo Z, Su W, Allen S, Pang H, Daugherty A, Smart E, Gong MC. COX-2 up-regulation and vascular smooth muscle contractile hyper-reactivity in spontaneous diabetic mice. Cardiovascular Research. 2005;67:723-35.
.
View Article PubMed Google Scholar -
Szerafin T, Erdei N, Fülöp T, Pasztor ET, Edes I, Koller A, Bagi Z. Increased cyclooxygenase-2 expression and prostaglandin-mediated dilation in coronary arterioles of patients with diabetes mellitus. Circulation Research. 2006;99:e12-7.
.
View Article PubMed Google Scholar -
Redondo S, Ruiz E, Gordillo-Moscoso A, Navarro-Dorado J, Ramajo M, Rodríguez E, Reguillo F, Carnero M, Casado M, Tejerina T. Overproduction of cyclo-oxygenase-2 (COX-2) is involved in the resistance to apoptosis in vascular smooth muscle cells from diabetic patients: a link between inflammation and apoptosis. Diabetologia. 2011;54:190-9.
.
View Article PubMed Google Scholar -
Cheng H, Fan X, Moeckel GW, Harris RC. Podocyte COX-2 exacerbates diabetic nephropathy by increasing podocyte (pro) renin receptor expression. Journal of American Society of Nephrology. 2011;22:1240-51.
.
View Article PubMed Google Scholar -
Cheng HF, Wang CJ, Moeckel GW, Zhang MZ, McKanna JA, Harris RC. Cyclooxygenase-2 inhibitor blocks expression of mediators of renal injury in a model of diabetes and hypertension. Kidney International. 2002;62:929-9.
.
View Article PubMed Google Scholar -
Nasrallah R, Robertson SJ, Hébert RL. Chronic COX inhibition reduces diabetes-induced hyperfiltration, proteinuria, and renal pathological markers in 36-week B6-Ins2Akita mice. American Journal of Nephrology. 2009;30:346-53.
.
View Article PubMed Google Scholar -
Quilley J, Santos M, Pedraza P. Renal protective effect of chronic inhibition of COX-2 with SC-58236 in streptozotocin-diabetic rats. American Journal of Physiology-Heart and Circulatory Physiology. 2011;300:H2316-22.
.
View Article PubMed Google Scholar -
Abdelrahman AM, Al Suleimani YM. Four-week administration of nimesulide, a cyclooxygenase-2 inhibitor, improves endothelial dysfunction in the hind limb vasculature of streptozotocin-induced diabetic rats. Archives of Pharmacal Research. 2008;31:1584-9.
.
View Article PubMed Google Scholar -
Botting RM. Inhibitors of cyclooxygenases: mechanisms, selectivity and uses. Journal of Physiology and Pharmacology. 2006;57:113-24.
.
PubMed Google Scholar -
Bunimov N, Laneuville O. Cyclooxygenase inhibitors: instrumental drugs to understand cardiovascular homeostasis and arterial thrombosis. Cardiovascular and Hematological Disorders-Drug Targets. 2008;8:268-77.
.
View Article Google Scholar -
Liavonchanka A, Feussne I. Lipoxygenases: Occurrence, functions and catalysis. Journal of Plant Physiology. 2005;163:348-35.
.
View Article Google Scholar -
Dobrian AD, Morris MA, Taylor-Fishwick DA, Holman TR, Imai Y, Mirmira RG, Nadler JL. Role of the 12-lipoxygenase pathway in diabetes pathogenesis and complications. Pharmacology and Therapeutics. 2019;195:100-10.
.
View Article Google Scholar -
Wei R, Enaka M, Muragaki Y. Activation of KEAP1/NRF2/P62 signaling alleviates high phosphate-induced calcification of vascular smooth muscle cells by suppressing reactive oxygen species production. Scientific Reports. 2019;9:10366.
.
View Article PubMed Google Scholar -
Luci D, Jameson JB, Yasgar A, Diaz G, Joshi N, Kantz A, Markham K, Perry S, Kuhn N, Yeung J, Schultz L, Holinstat M, Nadler J, Taylor-Fishwick DA, Jadhav A, Simeonov A, Holman TR, Maloney DJ. Discovery of ML355, a potent and selective inhibitor of human 12-lipoxygenase. [Updated 2014 Sep 18]. In: Probe Reports from the NIH Molecular Libraries Program [Internet]. Bethesda (MD): National Center for Biotechnology Information (US); 2010-. 2013.
.
View Article Google Scholar -
Adili R, Tourdot BE, Mast K, Yeung J, Freedman JC, Green A, Luci DK, Jadhav A, Simeonov A, Maloney DJ, Holman TR, Holinstat, M. First selective 12-LOX inhibitor, ML355, impairs thrombus formation and vessel occlusion in vivo with minimal effects on hemostasis highlights. Arteriosclerosis Thrombosis and Vascular Biology. 2017;37:1828-39.
.
View Article Google Scholar -
Rabbani N, Thornalley PJ. Hexokinase-2 glycolytic overload in diabetes and ischemia-reperfusion injury. Trends Endocrinology and Metabolism. 2019;30:419-31.
.
View Article Google Scholar -
Li W, Huang C, Hsieh Y, Chen T, Cheng L, Chen C, Liu C, Chen H, Huang C, Lo J, Chang K. Regulatory role of hexokinase 2 in modulating head and neck tumorigenesis. Frontiers in Oncology. 2020;10:176.
.
View Article Google Scholar -
Nederlof R, Gürel-Gurevin E , Eerbeek O, Xie C, Deijs GS, Konkel M, Hu J, Weber NC, Schumacher CA, Baartscheer A, Mik EG, Hollmann MW, Akar FG, Zuurbier CJ. Reducing mitochondrial bound hexokinase II mediates transition from non-injurious into injurious ischemia/reperfusion of the intact heart. Journal of Physiology and Biochemistry. 2017;73:323-33.
.
View Article PubMed Google Scholar -
Hariton F, Xue M, Rabbani N, Fowler M, Thornalley PJ. Sulforaphane delays fibroblast senescence by curbing cellular glucose uptake, increased glycolysis, and oxidative damage. Oxidative Medicine and Cellular Longevity. 2018;2018:5642148.
.
View Article PubMed Google Scholar -
Irshad Z, Xue M, Ashour A, Larkin JR, Thornalley PJ, Rabbani N. Activation of the unfolded protein response in high glucose treated endothelial cells is mediated by methylglyoxal. Scientific Reports. 2019;9:7889.
.
View Article PubMed Google Scholar -
Sans CL, Satterwhite DJ, Stoltzman CA, Breen KT, Ayer DE. MondoA-Mlx heterodimers are candidate sensors of cellular energy status: mitochondrial localization and direct regulation of glycolysis. Molecular and Cell Biology. 2006;26:4863-71.
.
View Article PubMed Google Scholar
Comments
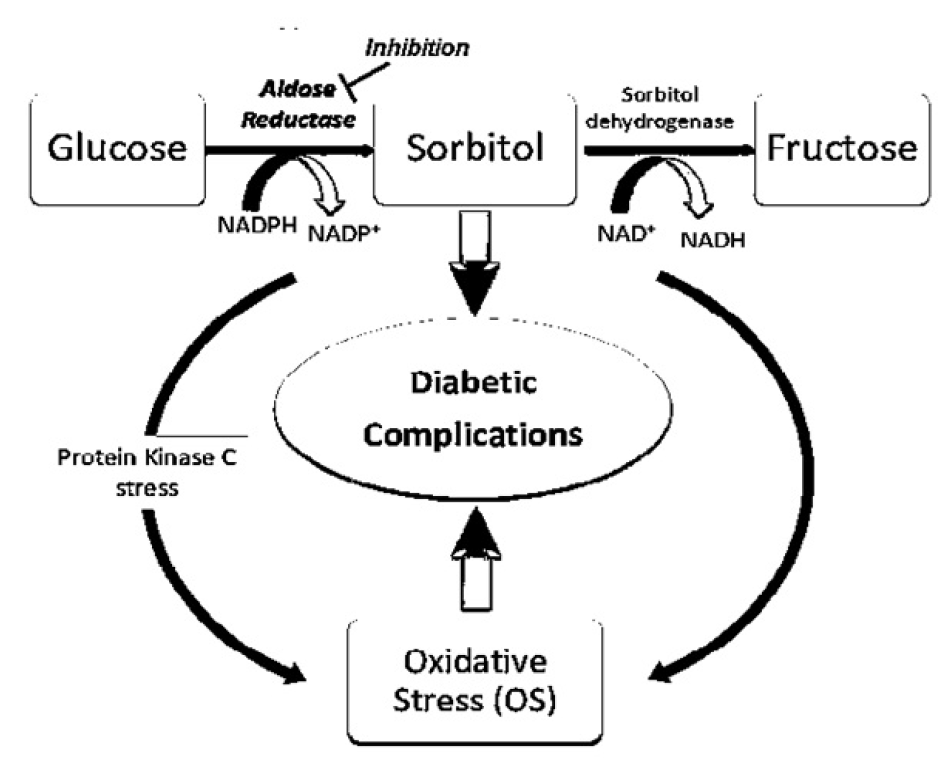
Downloads
Article Details
Volume & Issue : Vol 8 No 3 (2021)
Page No.: 4243-4257
Published on: 2021-03-31
Citations
Copyrights & License

This work is licensed under a Creative Commons Attribution 4.0 International License.
Search Panel
Pubmed
Google Scholar
Pubmed
Google Scholar
Pubmed
Search for this article in:
Google Scholar
Researchgate
- HTML viewed - 72232 times
- View Article downloaded - 0 times
- Download PDF downloaded - 8134 times