Abstract
Breast cancer is the world's most common cancer in women and is the leading cause of their cancer-related mortality. Its early diagnosis with conventional therapies such as surgery, chemotherapy, and radiotherapy can give good results in most breast cancer patients. However, these therapies provide poor outcomes in metastatic breast cancers or late-stage breast cancer. Therefore, as another effort for breast cancer treatment, immunotherapy is now considered the fourth-line cancer treatment besides conventional therapies. In this article, we focus on breast cancer treatment by transplantation of cytokine-induced killer cells (CIKs) and dendritic cells (DCs). While CIKs are effector cells that can directly attack and kill breast cancer cells, DCs support other immune cells in including CIKs in antitumor activities. Although transplantation of CIKs or DCs alone gave limited results in breast cancer treatment, the combination of CIKs and DCs in current clinical trials demonstrated significant results. Thus, we propose that CIK-DC therapy will emerge as a new option for breast cancer treatment soon.
Introduction
Breast cancer is the world's most common cancer in women and is the leading cause of their cancer-related mortality. It can usually affect women of all ages. In the US itself, breast cancer incidence in women is up by 30%, recorded in 20191. During 1996 – 2015, about 14,222 new breast cancer cases were reported (including 13,948 women, accounting for 98%), and more than half were diagnosed with stage II while stage III and IV were about 26%2.
Early diagnosis of breast cancer combined with conventional therapy such as surgery, chemotherapy, and radiotherapy is the most common strategy. However, due to the heterogeneous nature of breast cancer and the incidence of metastasis, it remains incurable. Therefore, in the efforts against cancer, immunotherapy has emerged as the fourth line of cancer treatment besides conventional therapy. Immunotherapy harnesses the complexity of the natural immune system to fight cancer, either actively or passively; the strategies aim to boost host immunity to fight cancer again. Massive research on immunotherapy has produced many promising clinical results, including treatment with checkpoint inhibitory, cytokine, and adoptive cell therapies3, 4. Additionally, the breakthrough of using anti-PD-1 and anti-PD-L1 antibodies in treatment with metastatic, triple-negative breast cancer patients has illuminated the field of immunotherapy for breast cancer treatment5.
Adoptive cell immunotherapy offers an approach that selectively targets cancer with high efficiency and low risk of side effects6. Cell immunotherapy is a promising strategy aimed at improving the antitumor activity of the immune system. Based on the concept of harnessing the immune system, several concepts have been developed for cell-based immunotherapy approach, including adoptive cell therapy with LAK, TIL, CAR-T, NK, and CIK or cancer vaccine with DCs-based immunotherapy.
In this review, we focus on studying cytokine-induced killer cells (CIK) and dendritic cells (DCs) in breast cancer treatment. Cell immunotherapy is a promising strategy aimed at improving the antitumor activity of an immune system.
Cytokine-induced killer cells & dendritic cells for breast cancer treatments
CIKs and their cytotoxic mechanisms toward tumor cells
What are CIKs
Cytokine-induced killer cells (CIKs) are a heterogeneous population characterized by the frequency of three populations: CD3+CD56+ (NK-like T), CD3+CD56- (T lymphocytes), and CD3-CD56+ (NK cells). This population is produced only by the in vitro culture of MNCs supplemented with cytokines. The first protocol for CIK production was introduced by Schmidt-Wolf et al. in the 1990s7.
How to produce CIKs?
CIK cells can be easily produced in ex-vivo conditions using MNCs from bone marrow, peripheral blood, or umbilical cord blood in combination with supplements of interferon-gamma (IFN- γ) and interleukin-2 (IL-2) with antibody-CD3 clone OKT3 over short-term of 2 – 3 weeks. The culture condition of CIK was modified from the LAK production protocol adding 1000 U/ml of INF- γ 24 hours before culture in the condition of anti-CD3 and IL-28. The addition of IFN-g significantly enhances CIK cytotoxicity compared to the LAK culture method. Indeed, IFN-g plays a role in inducing IL-12 production by activating monocytes9, 10. Furthermore, compared to LAK cells, CIK cells showed a higher ex-vivo expansion and prolonged in-vivo antitumor effect without exogenous cytokine IL-211, 12.
The ratios of the three different cell populations inside a CIK population are different between culture and inducible protocol. Generally, a CIK population is characterized by average 70 — 80% of CD3+ cells, CD3+CD8+ cells over 60–80% and CD3+CD56+ cells over 20 — 30%13. Antitumor effects of the CIK population are best seen in CD3+CD56+ population, a subset of CD3+ T lymphocytes that co-express natural killer cell protein CD5614, 15. The CD3+CD56+ subset is derived from CD3+CD8+ T lymphocytes, acquiring the terminally differentiated effector phenotype and granular structure of NK cells and higher levels of secreted antitumor cytokine IFN- γ, TNF-α, Granzyme B/Perforin15, 16, 17. In-vitro expanded-CIK significantly increased CD3+CD8+ T cells and CD3+CD56+ NK-like T cells15, 17.
Antitumor activity of CIK population
The antitumor activities of CIK population are acquired from the activities of three different cells inside. All subsets of CIK populations (CD3+CD56-, CD3+CD56+, and CD3-CD56+) display antitumor activity through various mechanisms (Figure 1).
CD3+CD56+ cell subset is capable of inducing MHC-unrestricted antitumor cytotoxicity14, 18. Indeed, CIKs also display their cytotoxic capacity in case of blocking of their receptors (CD2, CD3, CD8, CD28, CD56, very late antigen [VLA-4], T-cell receptor [TCR] αβ, MHC class I and II) by antibodies. The cytotoxic function of these populations heavily depends on engaging several activation receptors and releasing Granzyme-B/Perforin proteins from CIKs19. As a result of co-expression of NK and T cell markers, Pievani et al. (2011) suggested that the CD3+CD56+ cells acquire dual cytotoxic functions16, which stem from NK-cytotoxic functions and T-cell cytotoxic mechanisms. Antitumor activities of CIK cells require the direct interaction between CIK and tumor cells through surface markers16, 20. These interactions induce the release of granzyme B and perforin to mediate CIK-related killing function and promote IFN- γ and TNF-α production21. The interactions via receptors between CIKs and tumor cells are not well-understood; some recent studies suggested four main interactions between CIKs and tumor cells. The first interaction is performed by receptor leukocyte function-associated antigen-1 (LFA-1) on the CIKs with their ligands in tumor cells (ICAM-1, -2, and -3). Indeed, if the LFA-1 or ICAM-1 is blocked, the cytotoxic potential is significantly reduced15, 22, 23. The second interaction that plays an essential role in tumor recognition by CIK cells is the natural killer group 2 D (NKG2D) receptor on CIK cells and their ligands in tumor cells. NKG2D receptor is a member of the c-type lectin-activating receptor family expressed in the NK cells and NKG2D ligands: stress-inducible molecules on both solid and hematologic tumors, such as the MHC class I-related molecules A and B (MIC A/B) and members of the UL16-binding protein family (ULBP1-4) expressed in tumor cells. Interestingly, NKG2D ligands appear to express a pattern relatively restricted to malignant tumors24, 25. The expression of NKG2D receptors is involved in the high dose of IL-2 presenting in the culture medium. Besides IL-2, IL-15 also seems to be a target recognition of NKG2D26. The third interaction relates to the expression of CD56 expressed on CIKs with their ligands in tumor cells. Introna et al. suggested that CD56 plays a role in tumor recognition and cytotoxicity of CIK cells. Therefore, when antibodies blocked CD56 in the CIKs, the cytolysis of CIKs reduced27, 28. The fourth interaction relate to Fas ligand (FasL) highly expressed in CIKs and Fas on tumor cells8, 29. In a recent study, Meng et al. analyzed the transcriptomic atlas of CIKs and confirmed the high expression of FasL in CIKs30. The direct contact of FasL on Fas triggers Fas-dependent apoptosis mechanism in tumor cells15, 16, 19. Recently, the interactions of NKp30 and DNAM-1 expressed in CIKs with their ligands on tumor cells play a role in antitumor cytotoxicity of CIKs16.
Some recent studies revealed that CIK could perform the antibody-dependent cell cytotoxicity mediated by the expression of CD16. This observation is different between groups. Some studies suggested a subset of CD3+CD56+CD16+ in the population of CD3+CD56+ 31, 32, 33, 34, while other groups did not detect the expression of this protein in the CD3+CD56+ population11, 15, 35. Cappuzzello et al. suggested that the expression of CD16 in the CIK population was donor-dependent8. In a study of 60 samples, the CD3+CD56+CD16+ population ranged from 2.3% — 54.2% (mean 16 ± 13.3%). In vitro study and mAbs enhanced the specific lysis rate of CIK cells against EGFR- and Her-expressed cell lines. In TBNC-Patient-Derived Xenograft (PDX) models, treatment combining monoclonal antibody (mAb) and CIK significantly prolonged survival and reduced tumor volume. According to tumor section analyses, the combination also resulted in a higher infiltration of immune cells in the tumor36.
In-vivo antitumor activity of CIKs
In a preclinical study, infused-allogeneic CIK cells able to locate and increase in spleen and cervical lymph nodes and remain in tumor site for up to 21 suggested prolonged antitumor effects. In regard to graft versus host disease (GVHD), models, dose up to 20.106 allogeneic CIK cells was tolerated well compared to naïve T cell infusion, which quickly developed severe acute GVHD18. CIK cells demonstrated antitumor activity toward a wide range of cancer cell lines and freshly isolated cancer cells. Furthermore, numerous studies proved CIK's ability to treat both hematologic and solid tumors13, 20, 21, 37, 38. Recently, Capellero et al. published a preclinical study; the CIK cells from EOC patients efficiently killed patient-derived ovarian cancer cell lines (pdOVC), with no difference between autologous and allogeneic targets39. Also, the study indicated that CIK cells also efficiently killed chemotherapy-survived pdOVC; the killing ability was superior due to the high expression of stress ligand in tumor cells after being treated with carboplatin. In in-vivo models, CIK infusion resulted in high necrotic areas and a high rate of CIK infiltration. In a study with breast cancer cell line MCF-7, CIK strongly inhibits proliferation of both radio-resistant and normal MCF-7 cells17, 40.
Clinically, in 2020, Ying Zhang and Schmidt-Wolf published an updated international registry over the past ten years of CIK immunotherapy13. A total of 106 clinical trials was registered through IRCC; 4,889 patients with more than 30 types of cancers received CIK treatment along with/without conventional therapy. Treatment with CIK-based therapy significantly improves mPFS and mOS of patients. Patients’ immune system was significantly altered: CD3+CD56+, CD3+CD8+, CD4+/CD8+ population ratio were elevated while T-reg population CD4+CD25+FoxP3+ was decreased. Also, the level of Th-1 associated cytokine was increased after CIK treatment. In terms of safety, CIK treatment-related side effects were mostly grade I and II such as fever, chills, fatigue, headache, and skin rash. The incidence of grade III–IV toxicities was rare in the CIK treatment group. Infusion of allogeneic CIK was related to acute and chronic GVHD; however, patients showed good –tolerance the immunosuppressive regimen. The CIK treatment reported a higher Karnofsky score (KPS), better appetite, improved sleep, weight gain, and pain relief.
DCs and their cytotoxic mechanisms toward tumor cells
What dendritic cells are?
Dendritic cells (DCs) are known as professional antigen-presenting cells (APCs). The DCs’ ability to present antigen attracts attention as carriers for cancer vaccine approaches41. In the body, DCs are activated and matured in response to the environmental stimulator. The activation of DCs further mediates T cell activation through the engagement of MHC-class I/II and co-stimulation with cytokines. The DC-based vaccinations inhibit tumor growth by altering host lymphocyte composition. Ex-vivo expanded TAA-loaded DCs have been widely approached in a clinical study for targeting tumor and boosting specific-targeting immune response. Over the past two decades, DC-based therapy represents a feasible approach to elicit antitumor immunity while remaining safe and well-tolerated in patients.
How to produce DCs?
Current approaches of DC-based immunotherapy include the use of isolated CD14+ monocytes or CD34+ HPC from blood or bone marrow42. Several protocols have been developed using unstimulated DCs, ex-vivo matured DCs or cell-lysate/TAA-pulsed DCs, in-situ DC vaccination, and DC-derived exosomes43.
The first generation used tumor antigen-loaded immature DCs and achieved poor clinical response with only 3.3% tumor regression. The second-generation DC vaccines used matured monocyte-derived DCs, and the treatment reached 8 — 15% objective response rates with the median OS increasing by ~20% in some studies44.
The roles of DCs in anti-tumors
Unlike CIKs — effector cells that can directly attack cancer cells and kill them — DCs are antigen-presenting cells so that they indirectly strengthen the antitumor process of the immune system. However, they play an essential role in immune response in cancer treatment. Indeed, cancer cells usually escape from the immune surveillance in cancer patients, especially the sub-population of cancer stem cells inside. The effector T cells inside these patients cannot recognize cancer to kill them. DCs, in this case, will activate the T cells (both CD4+ and CD8+ cells). They can give some essential boost to immune responses in antitumor activity:
DCs enable CD4+ T cells to activate B and CD8+ cells. This process is based on the interaction between DCs and CD4+ T cells through CD40. The CD40 in DCs will interact with the CD40 ligand in T cells leading to DC activation. In the activated state, DCs can prime T cells and up-regulate the expression of some co-stimulatory molecules and produce IL-12. Then, IL-12 causes polarization in naïve CD4+ cells toward Th1 cells or Th2 cells. Th1 cells and Th2 cells will promote CD8+ cells and B cells through some cytokines (IL-2, IL-4, IL-5, IL-13, and IFN-g).
DCs also cross-talk with NKs and play a pivotal role in the innate immune response against cancer45. DCs interact with NKs via CXCR3 in the draining lymph nodes in a "touch and go" mode lasting from 300s to 4h46. As a result, DCs will produce IL-12, IL-18, IL-27, type I IFNs, and IL-15, PGE2. These cytokines directly affect NK cells, triggering NK cell proliferation and activating NK cells. The activated NK cells leave the lymph node, infiltrate tumors, and attack cancer cells in the tumors.
In-vivo antitumor activity of DCs
Clinical trials of DC vaccination showed promising results. In the role of APC, DCs are used to present tumor antigens to other immune cells. Therefore, both tumor-specific antigens and tumor-associated antigens are used in DC vaccinations. These antigens can be peptides/proteins, mRNA, or tumor lysates47. Thus, DC vaccination appears a safe and feasible strategy; furthermore, the vaccination combines with antigen-specific CTL activity and positive natural killer response in > 50% cancer patients48, 49.
In 2010, sipuleucel-T, the first cellular-based immunotherapy, was approved by USA FDA for the treatment of prostate cancer patients. The intervention was activated DCs by recombinant fusion protein PA2024, the fusion of GM-CSF with prostate antigen, which can be classified as the intersection between first and second generation of DC vaccines. The randomized clinical trial was conducted on 512 patients; sipuleucel-t treatment increased median survival by 4.1 months compared to the placebo group (25.8 months vs. 21.7 months, respectively); however, the treatment failed to achieve better disease progression50. Various kinds of cancer also were clinically treated by DCs such as glioblastoma51, 52, acute myeloid leukemia53, 54, breast cancer55, metastatic colorectal cancer56, prostate cancer57, mesothelioma58, lung cancer59, hepatocellular carcinoma60, pancreatic cancer61, advanced melanoma62, non-small cell lung cancer63, bone and soft tissue sarcoma64, and myeloma65, 66.
Collaborative mechanisms of CIKs and DCs in antitumor cells
Since DC therapy aims to improve host adaptive immune responses, different strategies have been developed harnessing the immune-stimulation effects of DC with effector cell-based immunotherapy ex vivo. Stimulation activity of DCs is through the ability to capture, processing and presenting a tumor-associated antigen (TAAs), which induces specific antitumor responses. The intervention combining DCs and T cells ex vivo resulted in a lower risk of relapse and metastasis, lower level of T-reg, and increased Th1 polarization in breast cancer patients67.
In recent years, several studies have reported that the synergistic antitumor effect of CIK blends with DCs13, 21, 68. The strategy provides the ability to target cancer cells in an MHC-independent manner through CIK cells, while DCs mount an immune response through an MHC-restriction mechanism. Co-culture of CIK and DCs significantly improved the antitumor effect by increasing cytokine IL-12 and IFN-g production; the interaction is a TCR-independent mechanism29, 69, 70. Recent studies have proved that DC enhances CIK through cell-cell contact in an MHC-independent manner but by CD40L/CD40; inhibiting CD40L/CD40 interaction abrogates these changes70, 71. Interaction of DCs and CIK thus alters the expression of several membrane proteins, including upregulation of membrane protein CD28 and CD40L on CIK, which are co-stimulatory signals promoting immune activation. Further, an increase in proliferation and CIK phenotype (including CD3+, CD8+, and CD3+CD56+ population) has been observed after co-culture with DCs72. The concomitant of Treg in CIK population also decreases in both cell and mRNA levels after co-culture with DCs72, 73. In in-vivo models, DC plus CIK revealed a superior antitumor effect compared to single therapy74. The treatment altered host immunity, altering host immune-system composition and augmenting immune antitumor response by enhancing CTL and NK-cell function. The ratio of CD4+/CD8+ significantly increased after DC/CIK treatment, represented the immunomodulatory effect, and improved immune-surveillance of DC-CIK to the host immune system75, 76. Also, the level of Th1-associated cytokine was elevated, including IL-2, IFN-g, IL-12. In reverse, the treatment resulted in a lower proportion of immunosuppressive factors, T-reg cell, cytokine IL-10, and TGF-β77, 78.
DCs plus CIKs have been proved as a promising immunotherapy approach in treatment of advanced solid tumors. In a ten-year review, 37 of 85 studies were conducted with DC-CIK treatment for lung cancer, hepatocellular carcinoma, pancreatic cancer, colorectal cancer, renal cell carcinoma, and breast cancer13. DC-CIK treatment shows significantly enhanced response rate in patients, better clinical benefit rate, and higher median overall survival compared to conventional treatment group.
Year (Ref) | Study type | Disease | Patients (treatment) | Pre-treatment | Intervention | Immunotherapy clinical response |
---|---|---|---|---|---|---|
2014 79 | Retrospective | TNBC (stage I-III) | 90 (45) | Surgery, adjuvant chemotherapy with or without radiation | autologous CIK cells 8.7 x 10 9 – 1.2 x 10 10 cells/cycle (4 - 52 cycles) | 1-, 2-, 3-, and 4-year DFS rate: 97.7%, 90.1%, 83.4%, 75.2%. 1-, 2-, 3-, and 4-year OS rate: 100.0%, 100.0%, 96.7%, 92.4%, |
2018 80 | Retrospective | TNBC | 340 (77) | Surgery, chemotherapy | autologous CIK cells 5 - 7.7 x 10 9 cells/cycle (1 - 19 cycles) | 5-years OS rate 94.3% 5-year DFS rate 77.9% PD: 16/77 cases (20.8%) Death: 4/77 cases (5.2%) |
2019 17 | Retrospective | BC stage I-III | 310 | Surgery with chemotherapy or radiotherapy or endocrino-therapy | autologous CIK 8.7 - 12 x 10 9 cells/cycle (at least 4 cycles) | 5-year OS rate: 85.7% 5-year RFS rate: 80.8% |
2019 81 | Retrospective | TBNC | 294 | Surgery and chemotherapy | autologous CIK > 5 x 10 9 cells/cycle ( 1 - 26 cycles) | 1-, 3-, and 5-year DFS rate: 99.3%, 91.8%, 99.1% 1-, 3-, and 5-year OS rate 99.3%, 96.6%, 93.4% |
2015 82 | Clinical trial | Metastatic breast cancer | 20 | Chemotherapy | autologous DC and CIK 1 x 10 9 CIK with 1 x 10 7 DCs per cycle (8 cycles) | CR: 3/20 cases PR: 12/20 cases SD: 2/20 cases PD: 3/20 cases |
2013 83 | Randomized Controlled Trial | Metastatic breast cancer | 166 (87) | Chemotherapy | HDC with autologous DC/CIK (3 cycles) | mOS: 33.1 months 3-year OS rate: 20.7% (18/87 patients). 4-year OS rate: 9.2% (8/87 cases) |
2015 84 | Clinical trial | Advanced cancer stage IV | 12 cases with breast cancer | Surgery or chemotherapy or radiation | autologous DC/CIK 5.7 + 2.94 x 10 9 cells/cycle (6 cycles) | DCR: 25% (3/12 cases) |
2016 85 | Clinical trial | TNBC | 23 | Chemotherapy | autologous DC/CIK (3 cycles) | PR: 3/23 SD: 56.5% (13/23) PD: 30.4% (7/23) ORR: 13% DCR: 69.6% mPFS was 13.5 months |
2017 86 | Retrospective | Stage IV brast cancer | 368 (188) | Chemotherapy | autologous DC/CIK 6 - 10 × 10 9 cells/infusion (4 infusions/cycle, > 3 cycles) | 5-year DFS rate: 42% 5-year OS rate: 44% |
Year | Identifier | Phase | Disease | Intervention | Status |
---|---|---|---|---|---|
2010 | NCT01232062 | not showed | Breast Neoplasms Neoplasm Metastasis | High dose chemotherapy with DC-CIK | Completed |
2011 | NCT01395056 | not showed | Breast Neoplasms Neoplasm Metastasis | Cyclophosphamide combined thiotepa and carboplatin chemotherapy combined with DC-CIK immunotherapy | Completed |
2015 | NCT02491697 | Phase 2 | Breast cancer | DC-CIK immunotherapy with capecitabine | Active, not recruiting |
2015 | NCT02539017 | Phase 2 | Triple Negative Breast Neoplasms | DC-CIK combined with chemotherapy | Withdrawn |
2015 | NCT02450357 | not showed | Neoplastic Cells, Circulating | DC-CIK immunotherapy | Completed |
2016 | NCT02886897 | Phase 1 Phase 2 | Breast Cancer | DC-CIK and anti-PD-1 antibody | Unknown |
2018 | NCT03524261 | Phase 2 | Advanced breast cancer | Activated CIK and CD3-MUC1 Bispecific Antibody | Withdrawn |
2020 | NCT04282044 | Phase 1 | Triple Negative Breast Cancer (advanced solid tumors) | CRX100 suspension (autologous CIK) | Recruiting |
2020 | NCT04476641 | Phase 2 | Breast cancer | DC-CIK immunotherapy | Recruiting |
Transplantation of CIKs and DCs to treat breast cancer
Transplantation of CIK cells in the treatment of breast cancer
The clinical approach of CIK-based adoptive cell therapy has been growing vigorously in recent years (Table 1, Table 2). In breast cancer treatment, many patients had enrolled in clinical retrospective studies of CIK immunotherapy.
In 2014, a retrospective study was published by Ke Pan et al.73; the study included 90 patients with TNBC status; 45 of them received adjuvant CIK immunotherapy (8.7 x 109 – 1.2 x 1010 cells/infusion) after completed chemotherapy without radiation therapy post-mastectomy. Following the CIK treatment, TNBC patients experienced better DFS and OS than conventional treatment, using The Kaplan–Meier survival analysis method (P = 0.0382 and P = 0.0046, respectively). The rate of 1-, 2-, 3-, and 4-year DFS was higher in the CIK treatment group (CIK-group: 97.7%, 90.1%, 83.4%, and 75.2%, respectively; control-group: 88.9%, 64.4%, 62.1%, and 56.4%, respectively). The rate of 1-, 2-, 3-, and 4-year OS was higher in CIK treatment group (CIK-group: 100.0%, 100.0%, 96.7%, and 92.4%, respectively; control-group: 95.6%, 88.6%, 76.3%, and 72.7%, respectively). In further analysis of prognosis in TNBC patients using Cox proportional hazards regression analyses, CIK treatment and disease status were significantly associated with favorable DFS and OS results. According to the Kaplan-Meier analysis result, CIK treatment significantly enhanced OS and DFS advanced-stage group. In contrast, the early-stage TNBC showed no significant difference in response to two treatment types73, 87. In 2019, a retrospective on 294 TNBC patients showed that CIK treatment significantly enhanced 1-, 3-, and 5-year DFS (P = 0.047) and OS rate (P = 0.007) compared to the control group (adjuvant chemotherapy w/o radiation)81. Furthermore, the data showed that higher CIK infusion was correlated with a better antitumor effect; more than six cycles of CIK treatment significantly improved DFS (P = 0.02) and OS (P = 0.04). A study on 77 CIK-treated patients similarly concluded that higher cycles (> 6) are associated with better prognosis (p = 0.002 in DFS, p = 0.024 in OS) and decreased risk of death87. CIK treatment lowered the incidence of metastasis, 16/147 patients in the CIK group compared to 29/147 patients in the control. In the univariate and multivariate analysis, CIK treatment influenced DFS and OS in patients; adjuvant CIK treatment was an independent prognostic factor for both DFS (HR = 0.520, 95% CI:0.271 – 0.998, P = 0.049) and OS (HR = 0.414, 95% CI:0.190 – 0.903, P = 0.027) in multivariate analysis. In a study of 310 postoperative breast cancer patients, patients were selected via random table method for the control and the CIK treatment group. The 5-year recurrence-free survival (RFS) rate and the 5-year OS rate were higher in the CIK treatment group than the control (17). In sub-group analysis according to disease type, patients with ER/PR+ and HER2- significantly benefited from CIK treatment, and significantly prolonged OS was reported. TNBC patients and ER+/PR+/HER2- patients also showed improved prognosis factors; however, those groups were not statistically different. The study found that PD-L1 positive patients experienced better CIK treatment response than PD-L1 negative patients did; significantly higher 5-year RFS (87.6% versus 76.4%, P = 0.048) and 5-year OS (95.2% versus 77.1%, P = 0.048%) was reported. This effect was reversed in the control treatment group; PD-L1 was correlated with worsened clinical outcomes. Further, negative PD-L1 patients in two cohorts did not statistically differ in RFS and OS, thus suggesting the use of PD-L1 as a biomarker for the adoptive immunotherapy approach for breast cancer patients.
Side effect in these retrospective studies was reported mostly as spontaneous fever; no intolerable or severe side effect was recorded following CIK treatment. Furthermore, no statistical difference was observed in the incidence of adverse effects between the two treatment groups.
Transplantation of DCs in treatment of breast cancer
In breast cancer treatment, HER-2/neu pulsed DC induced the expression of co-stimulator CD28 on CD8+ T cells in HER-2/neu+ DCIS patients88. Additionally, the treatment increased levels of Th1-cytokine IFN-g and induced HER-2/neu-specific CD8+ T cells with a lower level of inhibitory B7 ligand CTLA-4. A high disease-free survival rate and prolonged median disease-free survival were achieved in the vaccinated group, compared to control treatment89. P53-pulsed DC vaccinations showed p53-specific T cell response in advanced breast cancer patients90. Patients experienced prolonged survival and temporary regression of metastasis while no toxicity was observed during DC administration. ELISpot analyses analyzed the specific T cell response; some patients experienced stable disease and lymph node regression. Overall, the clinical efficiency of DC immunotherapy remains below expectations. Poor clinical outcomes result from several factors, including lack of appropriate target antigens, downregulation of TAA and MHC molecules in tumor cells, poor homing ability of adoptive transferred-DC to lymph node, and rate of inducing target-specific CTL and immune-suppressive tumor microenvironment42. However, DC vaccination is a safe approach, thus facilitating further modifications and research to improve the clinical results.
DC-CIK cell transplantation in treatment with breast cancer
Preclinical studies have proved the superior antitumor effects of DC-combined CIK70, 74, 91, 92. DC-CIK combination has been widely used in clinical trials besides chemotherapy for cancer treatment75, 93, 94, 95, 96. Co-culturing DC and CIK leads to greater CIK anti-cancer effect against cancer cell. In clinical trials, the DC-CIK combination also showed that the clinical response outweighs conventional therapy. In five accessed clinical studies on DC and CIK application, a total of 589 breast cancer patients were enrolled, including 330 patients under DC-CIK treatment. In 2013, Ren J et al. investigated the effect of high-dose chemotherapy and DC-CIK compared to standard dose chemotherapy for metastatic breast cancer treatment in 166 patients83. The intervention was two cycles of 120 mg/m2 docetaxel plus 175 mg/m2 thiotepa in combination with DC-CIK; the addition of carboplatin was optional. The trial group achieved a significantly higher objective response rate compared to SDC treatment, 25.9% versus 10.1%, respectively (P = 0.009). In summary, 2 CR cases (2.4%), 20 PR (23.5%) cases, and 42 SD (49.4%) cases were reported in the HDC+DC-CIK treatment group. The median-OS were double that of the control group, 33.1 months in the experiment group and 15.2 months in the control group (P < 0.001). The median-PFS also significantly improved in the experiment group, with an average of 10.2 months vs. 3.7 months (P < 0.001). In the Cox regression model, HDC plus DC-CIK treatment for HER-2 positive patients with less than three metastasis sites were correlated with better OS and PFS prognosis. In the following clinical study in 2016, 23 metastatic pre-treated TNBC patients received a combination of cyclophosphamide, thiotepa, carboplatin, and DC-CIK immunotherapy. The study reported a 13.0% objective response rate (3 PR cases) and a 69.6% disease control rate (3 PR cases, 13 SD cases). The median-FPS reached 13.5 months (95% CI, 10.1 – 16.9 months), and the median OS was 15.2 months (95% CI, 12.5 – 18.1 months)85. In 2017, Lin M et al. published a 10-year follow-up study from 2003–2013. About 368 staged-IV breast cancer patients were recruited, and 188 patients were treated with one cycle of low-dose chemotherapy (Carmofure) and at least three cycles of four DC-CIK infusions86. One infusion regimen included 6 – 9.109 DC-CIK cells in 250 ml saline plus 1500 U/ml IL-2 and 1% human albumin intravenously. Lymphocyte count and function were tested after DC-CIK treatment from chemotherapy treatment. Th1-type cytokine was elevated upon DC-CIK treatment, including IL-2, TNF-β, and INF- γ. DC-CIK treatment significantly improved OS and DFS compared to the control group. The 5-year DFS was 42% in the experiment group, while that of control group was 30% (P < 0.01). The 5-year OS was 44% in the experimental group versus 29% in the control group (P < 0.01). Additionally, DC-CIK treatment independently lowered the risk of disease progression (OR = 0.09, 95% CI 0.02 – 0.42, P < 0.01) and risk of death (OR = 0.05, 95% CI 0.01 – 0.37, p < 0.01), according to multivariate Cox proportional regression analysis. DC-CIK treatment represented a feasible cancer treatment strategy with minimal side effects. The most common side effects were related to the chemotherapy. No lethal adverse effects were reported following DC-CIK treatment. Patients have received at least one infusion of DC-CIK; no dose modification or disruption was reported. The most common side effect was fever.
In 2014, a meta-analysis study about DCs, CIKs, and the combination of DC-CIK treatment for breast cancer patients was published by Wang et al.97. The meta-analysis study was conducted from 27 clinical trials with 633 enrolled breast cancer patients and compared DCs and CIKs treatment versus non-DC/CIK treatment. According to the analyzed result, the 1-year survival rate of patients in the group was significantly improved (P < 0.0001) for DC/CIK treatment group. Higher rates of 2- and 3-year survival were also reported following DC-CIK treatment; however no significant statistical difference was noted between the two groups (2-year survival: 83% versus 76%, P = 0.07; 3-year survival: 64% versus 48%, P = 0.07). The Karnofsky Performance Status Scale (KPS) results showed that breast cancer patients significantly improved from DC-CIK treatment compared to non-DC-CIK therapy (OR: 12.40, 95% CI = 6.61-18.19, P < 0.0001). A higher clinical benefit rate was recorded in DC-CIK group; however, the data was not statistically different. Additionally, the study analyzed host immune response to DC-CIK therapy. Significantly increased proportion of CD3+, CD4+, CD16+, CD4+CD8+, CD3+CD56+ immune cell subsets (P < 0.00001) and enhancement in T cell immunity function (AG-NOR: OR = 0.68, P < 0.0001) were observed after DC-CIK treatment. Several antitumor response cytokines were elevated following DC-CIK treatment, including IL-2, IL-6, IL-12, IFN- γ, and TNF-α (P < 0.00001). Moreover, the level of serum cancer markers was significantly decreased after DC-CIK treatment. Later, Hu et al.95 published a meta-analysis to compare the efficacy and safety of DC-CIK therapy versus conventional chemotherapy for breast cancer treatment. The study was conducted based on 11 randomized clinical trials with 941 breast cancer patients (including 386 cases who experienced CIK or DC-CIK, 361 cases with conventional chemotherapy only), with no statistical difference between the two groups of patients. Most studies (9/11 studies) reported CR and PR; the difference was significant between the CIK-DC treatment group and the conventional treatment group (CR: RR = 1.54, 95% CI: 1.09-2.19; PR: RR = 1.33, 95% CI: 1.11 – 1.59). In the meta-analysis, ORR was reported in 10 studies, significantly different between DC-CIK and conventional groups (RR = 1.37, 95% CI: 1.20 – 1.57). The incidence of side effects was not significant between DC-CIK treatment and non-DC-CIK and conventional treatment groups in both meta-analyses. Side effects included fever, leucocyte decrease, gastrointestinal adverse effects (OR: 0.72, 95% CI: 0.36 – 1.45, P = 0.36)97 and leukopenia, thrombocytopenia, hair loss, nausea/vomiting, hepatic complications, and neurologic complications95.
Future perspectives
Current clinical trial data demonstrated that DC-CIK is a promising approach for breast cancer treatment21. With chemotherapy's success in improving clinical response, the combination of DC-CIK prolongs survival in breast cancer patients. In a recent study by Ren J et al. in 2013, DC-CIK combined with HDC was used as first-line treatment for metastatic breast cancer patients. Patients experienced delayed disease relapse and longer survival time83. Success in the clinical trials attracted research on CIK and DC; several strategies have been evaluated in vitro and in vivo. DC-CIK cells efficiently targeted cancer stem cells; autologous CIK cells inhibited tumor growth in PDX models91, 92, 98, 99, 100. Recent studies have focused on modified CIK cells with chimeric antigen receptors to enhance CIK cytotoxic function and cancer-targeting ability8, 101, 102. Ren et al. incorporated CIK cells with anti-EGFR chimeric antigen receptor (CAR); the CAR-CIKs showed superior antitumor target against EGFR-positive tumor cells103. The combination of CAR and CIK further enhanced the secretion of IL-2 and IFN-gamma by CIK cells. Besides aiming to modify CIK cells, the combination of CIK cells with commercial immunotherapy drugs is also under investigation. The combination of mAbs with CIK cells showed promising results in preclinical studies34, 36, 104. It improved cytotoxicity of CIK cells via ADCC and increased infiltrated CIK cells in tumor specimens. The prospective study by Zhou et al. showed that PD-L1 expression in TNBC patients correlated with better response to CIK treatment, thus suggesting the combination of PD-L1/PD-1 immunotherapy treatment with CIK cells17.
In addition to the traditional autologous approach, among several cell-based immunotherapies, CIK cells are suggested as potential allogeneic cellular immunotherapy capable of approaching an "off-the-shelf" strategy (Figure 2). The preclinical trial had demonstrated low GVHD ability of CIK cells: allograft of CIK cells associated with graft-versus-tumor (GvT) showed minimal graft-versus-host-disease (GvHD) side effect14, 18, 19, 105. The clinical trials showed that allogeneic CIKs after hematopoietic stem cell transplantation (HSCT) showed a low incidence of GvHD in recipients while inducing antitumor response106, 107, 108. In 2012, Linn et al. reported a clinical trial phase I/II with allogeneic-HSCT relapsed patients; in five patients who developed immune responses attributed to CIK cell infusion, the risk of acute-GvHD was low (3/16 patients) and easily controlled107. In the combination of allogeneic CIK with donor lymphocyte infusion (DLI), the incidence of a GVHD was mostly associated with DLI (8 of 12 cases, total 16%)109.
Moreover, umbilical cord blood is an abundant and available source of precursor cells for CIK; more cord blood cells exert low immunogenicity110, 111. The UCB-CIK cells showed greater proliferation capacity, lower immunogenicity, lower expression of inhibitory receptor PD-1, and less susceptibility to chemotherapy than PB-CIK cells do. Additionally, the UCB-CIK cells showed higher production of IFN- γ and IL-2 compared to PB-CIK cells. The antitumor effect was also higher in the UCB-CIK treatment group both in vitro and in vivo112. The clinical study further demonstrated the antitumor potential of UCB-CIK with minimal toxicities113, 114.
Further, large-scale production of GMP-grade CIK is under vigorous study; Castiglia S et al. and Palmerini P et al. suggested the significant impact of culture systems on CIK cell quality115, 116. Serum-free conditions were studied to abrogate the in-consistent quality of human serum and human pool plasma. A recent study demonstrated the uniformity of cryopreserved-CIK cells for up to one year117. Cryopreserved-CIK and cryopreserved PBMC derived-CIK maintained their cytotoxic function toward cancer cells, however, they were lower than freshly-cultured CIK cells117, 118.
Conclusion
In recent years, immunotherapies for breast cancer treatment have been developed vigorously. The success of DC and CIK in both preclinical and clinical studies demonstrated their position in first-line treatment. Besides, it is worth noting that DC and CIK cells are easily expanded in ex-vivo conditions in GMP with a high expansion rate compared to other adoptive cell therapies. Further, the use of CIK in a clinical trial is IL-2 independent, thus reducing the cytotoxicity of exogenous IL-2. Therefore, the treatment represents a promising approach with safety, tolerability, and minimal toxicity. In breast cancer treatment, the use of DC, CIK, or DC-CIK significantly prolonged the survival of patients, improved quality of life, and increased the patient's immunity function. However, the database was limited to China, the dosage of DC-CIK remained heterogeneous, and CIK cells’ function depended on donor quality. The clinical reports also showed inconsistent format and bias results. Therefore, it is essential to optimize the procedure of DC-CIK therapy to create standard criteria for evaluating DC-CIK. Furthermore, the DC-CIK therapy should be assessed in multi-centered studies on a larger scale and uniform patient disease status.
Abbreviations
CAR-T: Chimeric antigen receptor T cell
CIK: Cytokine induced killer cell
DFS: Disease-free survival
DLI: Donor lymphocyte infusion
GM-CSF: Granulocyte-macrophage colony-stimulatin factor
GvHD: Graft-versus-host-disease
GvT: graft-versus-tumor
HDC: High-dose chemotherapy
HSCT: Hematopoietic stem cell transplantation
ICAM: Intercellular cell adhesion molecule
IFN: Interferon
IL: Interleukine
LAK: Lymphokine-activated killer cell
MHC: Major histocompatibility complex
MIC A/B: MHC class I-related molecules A and B
NK: Natural killer cell
NKG2D: Natural killer group 2 D
OS: Overall survival
PB-CIK: Peripheral blood derived cytokine induced killer cell
PBMC: Peripheral blood mononucleated cell
RFS: Recurrence-free survival
TAAs: Tumor-associated antigen
TBNC: Triple-negative breast cancer
TCR: T-cell receptor
Th1: T helper cell 1
TIL: Tumor-infiltrating lymphocyte
T-reg: Regulatory T cell
UCB-CIK: Umbilical cord blood derived cytokine induced killer cell
VLA-4: Very late antigen 4
Acknowledgments
None.
Author’s contributions
All authors equally contributed in this work. All authors read and approved the final version of the manuscript for submission.
Funding
This research is funded by Vietnam National University Ho Chi Minh City (VNU-HCM) under grant number C2020-18-26.
Availability of data and materials
Not applicable.
Ethics approval and consent to participate
Not applicable.
Consent for publication
Not applicable.
Competing interests
The authors declare that they have no competing interests.
References
-
Siegel
R.L.,
Miller
K.D.,
Jemal
A.,
Cancer statistics, 2019. CA: a Cancer Journal for Clinicians.
2019;
69
(1)
:
7-34
.
View Article PubMed Google Scholar -
Pham
D.X.,
Ho
T.H.,
Bui
T.D.,
Ho-Pham
L.T.,
Nguyen
T.V.,
Trends in breast cancer incidence in Ho Chi Minh City 1996-2015: A registry-based study. PLoS One.
2021;
16
(2)
:
e0246800
.
View Article PubMed Google Scholar -
Waldman
A.D.,
Fritz
J.M.,
Lenardo
M.J.,
A guide to cancer immunotherapy: from T cell basic science to clinical practice. Nature Reviews. Immunology.
2020;
20
(11)
:
651-68
.
View Article PubMed Google Scholar -
Berraondo
P.,
Sanmamed
M.F.,
Ochoa
M.C.,
Etxeberria
I.,
Aznar
M.A.,
Pérez-Gracia
J.L.,
Cytokines in clinical cancer immunotherapy. British Journal of Cancer.
2019;
120
(1)
:
6-15
.
View Article PubMed Google Scholar -
Pusztai
L.,
Karn
T.,
Safonov
A.,
Abu-Khalaf
M.M.,
Bianchini
G.,
New Strategies in Breast Cancer: immunotherapy. Clinical Cancer Research.
2016;
22
(9)
:
2105-10
.
View Article PubMed Google Scholar -
Restifo
N.P.,
Dudley
M.E.,
Rosenberg
S.A.,
Adoptive immunotherapy for cancer: harnessing the T cell response. Nature Reviews. Immunology.
2012;
12
(4)
:
269-81
.
View Article PubMed Google Scholar -
Schmidt-Wolf
I.G.,
Negrin
R.S.,
Kiem
H.P.,
Blume
K.G.,
Weissman
I.L.,
Use of a SCID mouse/human lymphoma model to evaluate cytokine-induced killer cells with potent antitumor cell activity. The Journal of Experimental Medicine.
1991;
174
(1)
:
139-49
.
View Article PubMed Google Scholar -
Cappuzzello
E.,
Sommaggio
R.,
Zanovello
P.,
Rosato
A.,
Cytokines for the induction of antitumor effectors: the paradigm of Cytokine-Induced Killer (CIK) cells. Cytokine & Growth Factor Reviews.
2017;
36
:
99-105
.
View Article PubMed Google Scholar -
Trinchieri
G.,
Interleukin-12: a cytokine produced by antigen-presenting cells with immunoregulatory functions in the generation of T-helper cells type 1 and cytotoxic lymphocytes. Blood.
1994;
84
(12)
:
4008-27
.
View Article PubMed Google Scholar -
Lopez
R.D.,
Waller
E.K.,
Lu
P.H.,
Negrin
R.S.,
CD58/LFA-3 and IL-12 provided by activated monocytes are critical in the in vitro expansion of CD56+ T cells. Cancer Immunology, Immunotherapy.
2001;
49
(12)
:
629-40
.
View Article PubMed Google Scholar -
Lu
P.H.,
Negrin
R.S.,
A novel population of expanded human CD3+CD56+ cells derived from T cells with potent in vivo antitumor activity in mice with severe combined immunodeficiency. Journal of immunology (Baltimore, Md. : 1950).
1950;
153
:
1687-96
.
-
Linn
Y.C.,
Hui
K.M.,
Cytokine-induced killer cells: NK-like T cells with cytotolytic specificity against leukemia. Leukemia & Lymphoma.
2003;
44
(9)
:
1457-62
.
View Article PubMed Google Scholar -
Zhang
Y.,
Schmidt-Wolf
I.G.,
Ten-year update of the international registry on cytokine-induced killer cells in cancer immunotherapy. Journal of Cellular Physiology.
2020;
235
(12)
:
9291-303
.
View Article PubMed Google Scholar -
Sangiolo
D.,
Martinuzzi
E.,
Todorovic
M.,
Vitaggio
K.,
Vallario
A.,
Jordaney
N.,
Alloreactivity and anti-tumor activity segregate within two distinct subsets of cytokine-induced killer (CIK) cells: implications for their infusion across major HLA barriers. International Immunology.
2008;
20
(7)
:
841-8
.
View Article PubMed Google Scholar -
Franceschetti
M.,
Pievani
A.,
Borleri
G.,
Vago
L.,
Fleischhauer
K.,
Golay
J.,
Cytokine-induced killer cells are terminally differentiated activated CD8 cytotoxic T-EMRA lymphocytes. Experimental Hematology.
2009;
37
(5)
.
View Article PubMed Google Scholar -
Pievani
A.,
Borleri
G.,
Pende
D.,
Moretta
L.,
Rambaldi
A.,
Golay
J.,
Dual-functional capability of CD3+CD56+ CIK cells, a T-cell subset that acquires NK function and retains TCR-mediated specific cytotoxicity. Blood.
2011;
118
(12)
:
3301-10
.
View Article PubMed Google Scholar -
Zhou
Z.Q.,
Zhao
J.J.,
Pan
Q.Z.,
Chen
C.L.,
Liu
Y.,
Tang
Y.,
PD-L1 expression is a predictive biomarker for CIK cell-based immunotherapy in postoperative patients with breast cancer. Journal for Immunotherapy of Cancer.
2019;
7
(1)
:
228
.
View Article PubMed Google Scholar -
Nishimura
R.,
Baker
J.,
Beilhack
A.,
Zeiser
R.,
Olson
J.A.,
Sega
E.I.,
In vivo trafficking and survival of cytokine-induced killer cells resulting in minimal GVHD with retention of antitumor activity. Blood.
2008;
112
(6)
:
2563-74
.
View Article PubMed Google Scholar -
Baker
J.,
Verneris
M.R.,
Ito
M.,
Shizuru
J.A.,
Negrin
R.S.,
Expansion of cytolytic CD8(+) natural killer T cells with limited capacity for graft-versus-host disease induction due to interferon γ production. Blood.
2001;
97
(10)
:
2923-31
.
View Article PubMed Google Scholar -
Jiang
J.,
Wu
C.,
Lu
B.,
Cytokine-induced killer cells promote antitumor immunity. J Transl Med.
2013;
11
:
83
.
View Article PubMed Google Scholar -
Garofano
F.,
Gonzalez-Carmona
M.A.,
Skowasch
D.,
Schmidt-Wolf
R.,
Abramian
A.,
Hauser
S.,
Clinical Trials with Combination of Cytokine-Induced Killer Cells and Dendritic Cells for Cancer Therapy. International Journal of Molecular Sciences.
2019;
20
(17)
:
4307
.
View Article PubMed Google Scholar -
Schmidt-Wolf
I.G.,
Lefterova
P.,
Mehta
B.A.,
Fernandez
L.P.,
Huhn
D.,
Blume
K.G.,
Phenotypic characterization and identification of effector cells involved in tumor cell recognition of cytokine-induced killer cells. Experimental Hematology.
1993;
21
(13)
:
1673-9
.
PubMed Google Scholar -
Schmidt-Wolf
I.G.,
Lefterova
P.,
Johnston
V.,
Scheffold
C.,
Csipai
M.,
Mehta
B.A.,
Sensitivity of multidrug-resistant tumor cell lines to immunologic effector cells. Cellular Immunology.
1996;
169
(1)
:
85-90
.
View Article PubMed Google Scholar -
Jamieson
A.M.,
Diefenbach
A.,
McMahon
C.W.,
Xiong
N.,
Carlyle
J.R.,
Raulet
D.H.,
The role of the NKG2D immunoreceptor in immune cell activation and natural killing. Immunity.
2002;
17
(1)
:
19-29
.
View Article PubMed Google Scholar -
Diefenbach
A.,
Jamieson
A.M.,
Liu
S.D.,
Shastri
N.,
Raulet
D.H.,
Ligands for the murine NKG2D receptor: expression by tumor cells and activation of NK cells and macrophages. Nature Immunology.
2000;
1
(2)
:
119-26
.
View Article PubMed Google Scholar -
Rettinger
E.,
Kuçi
S.,
Naumann
I.,
Becker
P.,
Kreyenberg
H.,
Anzaghe
M.,
The cytotoxic potential of interleukin-15-stimulated cytokine-induced killer cells against leukemia cells. Cytotherapy.
2012;
14
(1)
:
91-103
.
View Article PubMed Google Scholar -
Introna
M.,
CIK as therapeutic agents against tumors. Journal of Autoimmunity.
2017;
85
:
32-44
.
View Article PubMed Google Scholar -
Valgardsdottir
R.,
Capitanio
C.,
Texido
G.,
Pende
D.,
Cantoni
C.,
Pesenti
E.,
Direct involvement of CD56 in cytokine-induced killer-mediated lysis of CD56+ hematopoietic target cells. Experimental Hematology.
2014;
42
(12)
.
View Article PubMed Google Scholar -
Zhang
Q.,
Liu
X.Y.,
Zhang
T.,
Zhang
X.F.,
Zhao
L.,
Long
F.,
The dual-functional capability of cytokine-induced killer cells and application in tumor immunology. Human Immunology.
2015;
76
(5)
:
385-91
.
View Article PubMed Google Scholar -
Meng
M.,
Li
L.,
Li
R.,
Wang
W.,
Chen
Y.,
Xie
Y.,
A dynamic transcriptomic atlas of cytokine-induced killer cells. The Journal of Biological Chemistry.
2018;
293
(51)
:
19600-12
.
View Article PubMed Google Scholar -
Karimi
M.,
Cao
T.M.,
Baker
J.A.,
Verneris
M.R.,
Soares
L.,
Negrin
R.S.,
Silencing human NKG2D, DAP10, and DAP12 reduces cytotoxicity of activated CD8+ T cells and NK cells. Journal of immunology (Baltimore, Md. : 1950).
2005;
175
:
7819-28
.
View Article Google Scholar -
Bonanno
G.,
Iudicone
P.,
Mariotti
A.,
Procoli
A.,
Pandolfi
A.,
Fioravanti
D.,
Thymoglobulin, interferon-γ and interleukin-2 efficiently expand cytokine-induced killer (CIK) cells in clinical-grade cultures. Journal of Translational Medicine.
2010;
8
(1)
:
129
.
View Article PubMed Google Scholar -
Thorne
S.H.,
Negrin
R.S.,
Contag
C.H.,
Synergistic antitumor effects of immune cell-viral biotherapy. Science.
2006;
311
(5768)
:
1780-4
.
View Article PubMed Google Scholar -
Cappuzzello
E.,
Tosi
A.,
Zanovello
P.,
Sommaggio
R.,
Rosato
A.,
Retargeting cytokine-induced killer cell activity by CD16 engagement with clinical-grade antibodies. Oncoimmunology.
2016;
5
:
e1199311-e
.
View Article Google Scholar -
Schmidt-Wolf
G.D.,
Negrin
R.S.,
Schmidt-Wolf
I.G.,
Activated T cells and cytokine-induced CD3+CD56+ killer cells. Annals of Hematology.
1997;
74
(2)
:
51-6
.
View Article PubMed Google Scholar -
Sommaggio
R.,
Cappuzzello
E.,
Dalla Pietà
A.,
Tosi
A.,
Palmerini
P.,
Carpanese
D.,
Adoptive cell therapy of triple negative breast cancer with redirected cytokine-induced killer cells. OncoImmunology.
2020;
9
(1)
:
1777046
.
View Article PubMed Google Scholar -
Gao
X.,
Mi
Y.,
Guo
N.,
Xu
H.,
Xu
L.,
Gou
X.,
Cytokine-Induced Killer Cells As Pharmacological Tools for Cancer Immunotherapy. Frontiers in Immunology.
2017;
8
:
774
.
View Article PubMed Google Scholar -
Li
X.D.,
Xu
B.,
Wu
J.,
Ji
M.,
Xu
B.H.,
Jiang
J.T.,
Review of Chinese clinical trials on CIK cell treatment for malignancies. Clinical & Translational Oncology.
2012;
14
(2)
:
102-8
.
View Article PubMed Google Scholar -
Capellero
S.,
Erriquez
J.,
Melano
C.,
Mesiano
G.,
Genta
S.,
Pisacane
A.,
Preclinical immunotherapy with Cytokine-Induced Killer lymphocytes against epithelial ovarian cancer. Scientific Reports.
2020;
10
(1)
:
6478
.
View Article PubMed Google Scholar -
Guo
Q.,
Zhu
D.,
Bu
X.,
Wei
X.,
Li
C.,
Gao
D.,
Efficient killing of radioresistant breast cancer cells by cytokine-induced killer cells. Tumour Biology.
2017;
39
(3)
:
1010428317695961
.
View Article PubMed Google Scholar -
Anguille
S.,
Smits
E.L.,
Bryant
C.,
Van Acker
H.H.,
Goossens
H.,
Lion
E.,
Dendritic Cells as Pharmacological Tools for Cancer Immunotherapy. Pharmacological Reviews.
2015;
67
(4)
:
731-53
.
View Article PubMed Google Scholar -
van Gulijk
M.,
Dammeijer
F.,
Aerts
J.G.,
Vroman
H.,
Combination Strategies to Optimize Efficacy of Dendritic Cell-Based Immunotherapy. Frontiers in Immunology.
2018;
9
:
2759
.
View Article PubMed Google Scholar -
Garg
A.D.,
Vara Perez
M.,
Schaaf
M.,
Agostinis
P.,
Zitvogel
L.,
Kroemer
G.,
Trial watch: dendritic cell-based anticancer immunotherapy. OncoImmunology.
2017;
6
(7)
:
e1328341
.
View Article PubMed Google Scholar -
Garg
A.D.,
Coulie
P.G.,
Van den Eynde
B.J.,
Agostinis
P.,
Integrating Next-Generation Dendritic Cell Vaccines into the Current Cancer Immunotherapy Landscape. Trends in Immunology.
2017;
38
(8)
:
577-93
.
View Article PubMed Google Scholar -
Harizi
H.,
Reciprocal crosstalk between dendritic cells and natural killer cells under the effects of PGE2 in immunity and immunopathology. Cellular & Molecular Immunology.
2013;
10
(3)
:
213-21
.
View Article PubMed Google Scholar -
Mingozzi
F.,
Spreafico
R.,
Gorletta
T.,
Cigni
C.,
Di Gioia
M.,
Caccia
M.,
Prolonged contact with dendritic cells turns lymph node-resident NK cells into anti-tumor effectors. EMBO Molecular Medicine.
2016;
8
(9)
:
1039-51
.
View Article PubMed Google Scholar -
Zhou
Y.,
Bosch
M.L.,
Salgaller
M.L.,
. J. 25: 289-303. doi: 10.1097/00002371-200207000-00001.
Current methods for loading dendritic cells with tumor antigen for the induction of antitumor immunity. 2002;
25
:
289-303
.
View Article Google Scholar -
Draube
A.,
Klein-González
N.,
Mattheus
S.,
Brillant
C.,
Hellmich
M.,
Engert
A.,
Dendritic cell based tumor vaccination in prostate and renal cell cancer: a systematic review and meta-analysis. PLoS One.
2011;
6
(4)
:
e18801
.
View Article PubMed Google Scholar -
Lion
E.,
Smits
E.L.,
Berneman
Z.N.,
Van Tendeloo
V.F.,
NK cells: key to success of DC-based cancer vaccines?. The Oncologist.
2012;
17
(10)
:
1256-70
.
View Article PubMed Google Scholar -
Kantoff
P.W.,
Higano
C.S.,
Shore
N.D.,
Berger
E.R.,
Small
E.J.,
Penson
D.F.,
Study Investigators
IMPACT,
Sipuleucel-T immunotherapy for castration-resistant prostate cancer. The New England Journal of Medicine.
2010;
363
(5)
:
411-22
.
View Article PubMed Google Scholar -
Reap
E.A.,
Suryadevara
C.M.,
Batich
K.A.,
Sanchez-Perez
L.,
Archer
G.E.,
Schmittling
R.J.,
Dendritic Cells Enhance Polyfunctionality of Adoptively Transferred T Cells That Target Cytomegalovirus in Glioblastoma. Cancer Research.
2018;
78
(1)
:
256-64
.
View Article PubMed Google Scholar -
Batich
K.A.,
Reap
E.A.,
Archer
G.E.,
Sanchez-Perez
L.,
Nair
S.K.,
Schmittling
R.J.,
Long-term Survival in Glioblastoma with Cytomegalovirus pp65-Targeted Vaccination. Clinical Cancer Research.
2017;
23
(8)
:
1898-909
.
View Article PubMed Google Scholar -
Khoury
H.J.,
Collins
R.H.,
Blum
W.,
Stiff
P.S.,
Elias
L.,
Lebkowski
J.S.,
Immune responses and long-term disease recurrence status after telomerase-based dendritic cell immunotherapy in patients with acute myeloid leukemia. Cancer.
2017;
123
(16)
:
3061-72
.
View Article PubMed Google Scholar -
Anguille
S.,
Van de Velde
A.L.,
Smits
E.L.,
Van Tendeloo
V.F.,
Juliusson
G.,
Cools
N.,
Dendritic cell vaccination as postremission treatment to prevent or delay relapse in acute myeloid leukemia. Blood.
2017;
130
(15)
:
1713-21
.
View Article PubMed Google Scholar -
Lowenfeld
L.,
Mick
R.,
Datta
J.,
Xu
S.,
Fitzpatrick
E.,
Fisher
C.S.,
Dendritic Cell Vaccination Enhances Immune Responses and Induces Regression of HER2pos DCIS Independent of Route: Results of Randomized Selection Design Trial. Clinical Cancer Research.
2017;
23
(12)
:
2961-71
.
View Article PubMed Google Scholar -
Caballero-Baños
M.,
Benitez-Ribas
D.,
Tabera
J.,
Phase II randomised trial of autologous tumour lysate dendritic cell plus best supportive care compared with best supportive care in pre-treated advanced colorectal cancer patients. European journal of cancer (Oxford, England : 1990).
2016;
64
:
167-74
.
View Article Google Scholar -
Podrazil
M.,
Horvath
R.,
Becht
E.,
Rozkova
D.,
Bilkova
P.,
Sochorova
K.,
Phase I/II clinical trial of dendritic-cell based immunotherapy (DCVAC/PCa) combined with chemotherapy in patients with metastatic, castration-resistant prostate cancer. Oncotarget.
2015;
6
(20)
:
18192-205
.
View Article PubMed Google Scholar -
Aerts
J.G.,
de Goeje
P.L.,
Cornelissen
R.,
Kaijen-Lambers
M.E.,
Bezemer
K.,
van der Leest
C.H.,
Autologous Dendritic Cells Pulsed with Allogeneic Tumor Cell Lysate in Mesothelioma: From Mouse to Human. Clinical Cancer Research.
2018;
24
(4)
:
766-76
.
View Article PubMed Google Scholar -
Kimura
H.,
Matsui
Y.,
Ishikawa
A.,
Nakajima
T.,
Iizasa
T.,
Randomized controlled phase III trial of adjuvant chemoimmunotherapy with activated cytotoxic T cells and dendritic cells from regional lymph nodes of patients with lung cancer. Cancer Immunology, Immunotherapy.
2018;
67
(8)
:
1231-8
.
View Article PubMed Google Scholar -
Lee
J.H.,
Lee
Y.,
Lee
M.,
Heo
M.K.,
Song
J.S.,
Kim
K.H.,
A phase I/IIa study of adjuvant immunotherapy with tumour antigen-pulsed dendritic cells in patients with hepatocellular carcinoma. British Journal of Cancer.
2015;
113
(12)
:
1666-76
.
View Article PubMed Google Scholar -
Mehrotra
S.,
Britten
C.D.,
Chin
S.,
Garrett-Mayer
E.,
Cloud
C.A.,
Li
M.,
Vaccination with poly(IC:LC) and peptide-pulsed autologous dendritic cells in patients with pancreatic cancer. Journal of Hematology & Oncology.
2017;
10
(1)
:
82
.
View Article PubMed Google Scholar -
Wilgenhof
S.,
Corthals
J.,
Heirman
C.,
van Baren
N.,
Lucas
S.,
Kvistborg
P.,
Phase II Study of Autologous Monocyte-Derived mRNA Electroporated Dendritic Cells (TriMixDC-MEL) Plus Ipilimumab in Patients With Pretreated Advanced Melanoma. Journal of Clinical Oncology.
2016;
34
(12)
:
1330-8
.
View Article PubMed Google Scholar -
Lee
J.M.,
Lee
M.H.,
Garon
E.,
Goldman
J.W.,
Salehi-Rad
R.,
Baratelli
F.E.,
Phase I Trial of Intratumoral Injection of CCL21 Gene-Modified Dendritic Cells in Lung Cancer Elicits Tumor-Specific Immune Responses and CD8+ T-cell Infiltration. Clinical Cancer Research.
2017;
23
(16)
:
4556-68
.
View Article PubMed Google Scholar -
Miwa
S.,
Nishida
H.,
Tanzawa
Y.,
Takeuchi
A.,
Hayashi
K.,
Yamamoto
N.,
Phase 1/2 study of immunotherapy with dendritic cells pulsed with autologous tumor lysate in patients with refractory bone and soft tissue sarcoma. Cancer.
2017;
123
(9)
:
1576-84
.
View Article PubMed Google Scholar -
Jung
S.H.,
Lee
H.J.,
Lee
Y.K.,
Yang
D.H.,
Kim
H.J.,
Rhee
J.H.,
A phase I clinical study of autologous dendritic cell therapy in patients with relapsed or refractory multiple myeloma. Oncotarget.
2017;
8
(25)
:
41538-48
.
View Article PubMed Google Scholar -
Butterfield
L.H.,
Zhao
F.,
Lee
S.,
Tarhini
A.A.,
Margolin
K.A.,
White
R.L.,
Immune Correlates of GM-CSF and Melanoma Peptide Vaccination in a Randomized Trial for the Adjuvant Therapy of Resected High-Risk Melanoma (E4697). Clinical Cancer Research.
2017;
23
(17)
:
5034-43
.
View Article PubMed Google Scholar -
Shevchenko
J.A.,
Khristin
A.A.,
Kurilin
V.V.,
Kuznetsova
M.S.,
Blinova
D.D.,
Starostina
N.M.,
Autologous dendritic cells and activated cytotoxic T cells as combination therapy for breast cancer. Oncology Reports.
2020;
43
(2)
:
671-80
.
View Article PubMed Google Scholar -
Wang
S.,
Wang
X.,
Zhou
X.,
Lyerly
H.K.,
Morse
M.A.,
Ren
J.,
DC-CIK as a widely applicable cancer immunotherapy. Expert Opinion on Biological Therapy.
2020;
20
(6)
:
601-7
.
View Article PubMed Google Scholar -
Joshi
P.S.,
Liu
J.-Q.,
Wang
Y.,
Chang
X.,
Richards
J.,
Assarsson
E.,
Shi
F.-D.,
Ljunggren
H.-G.,
Bai
X.-F.,
Cytokine-induced killer T cells kill immature dendritic cells by TCR-independent and perforin-dependent mechanisms. Cell Development, Growth, Differentiation, and Function.
2006;
80
:
1345-53
.
View Article Google Scholar -
Märten
A.,
Ziske
C.,
Schöttker
B.,
Interactions between dendritic cells and cytokine-induced killer cells lead to an activation of both populations. Journal of immunotherapy (Hagerstown, Md. : 1997).
2001;
24
:
502-10
.
View Article Google Scholar -
Lee
H.K.,
Kim
Y.G.,
Kim
J.S.,
Park
E.J.,
Kim
B.,
Park
K.H.,
Cytokine-induced killer cells interact with tumor lysate-pulsed dendritic cells via CCR5 signaling. Cancer Letters.
2016;
378
(2)
:
142-9
.
View Article PubMed Google Scholar -
Cao
J.,
Chen
C.,
Wang
Y.,
Chen
X.,
Chen
Z.,
Luo
X.,
Influence of autologous dendritic cells on cytokine-induced killer cell proliferation, cell phenotype and antitumor activity in vitro. Oncology Letters.
2016;
12
(3)
:
2033-7
.
View Article PubMed Google Scholar -
Pan
Y.,
Tao
Q.,
Wang
H.,
Xiong
S.,
Zhang
R.,
Chen
T.,
Dendritic cells decreased the concomitant expanded Tregs and Tregs related IL-35 in cytokine-induced killer cells and increased their cytotoxicity against leukemia cells. PLoS One.
2014;
9
(4)
:
e93591
.
View Article PubMed Google Scholar -
Jung
N.C.,
Lee
J.H.,
Choi
H.J.,
Hwang
S.U.,
Song
J.Y.,
Seo
H.G.,
Dendritic Cell Immunotherapy Combined with Cytokine-Induced Killer Cells Effectively Suppresses Established Hepatocellular Carcinomas in Mice. Immunological Investigations.
2016;
45
(6)
:
553-65
.
View Article PubMed Google Scholar -
Mu
Y.,
Zhou
C.H.,
Chen
S.F.,
Ding
J.,
Zhang
Y.X.,
Yang
Y.P.,
Effectiveness and safety of chemotherapy combined with cytokine-induced killer cell /dendritic cell-cytokine-induced killer cell therapy for treatment of gastric cancer in China: A systematic review and meta-analysis. Cytotherapy.
2016;
18
(9)
:
1162-77
.
View Article PubMed Google Scholar -
Xiao
Z.,
Wang
C.-Q.,
Zhou
M.-H.,
The Antitumor Immunity and Tumor Responses of Chemotherapy with or without DC-CIK for Non-Small-Cell Lung Cancer in China: A Meta-Analysis of 28 Randomized Controlled Trials. J Immunol Res.
2018;
2018
:
9081938
.
View Article Google Scholar -
Song
H.,
Liu
S.,
Zhao
Z.,
Sun
W.,
Wei
X.,
Ma
X.,
Increased cycles of DC/CIK immunotherapy decreases frequency of Tregs in patients with resected NSCLC. International Immunopharmacology.
2017;
52
:
197-202
.
View Article PubMed Google Scholar -
Zhao
X.,
Ji
C.Y.,
Liu
G.Q.,
Ma
D.X.,
Ding
H.F.,
Xu
M.,
Immunomodulatory effect of DC/CIK combined with chemotherapy in multiple myeloma and the clinical efficacy. International Journal of Clinical and Experimental Pathology.
2015;
8
(10)
:
13146-55
.
PubMed Google Scholar -
Ren
J.,
Di
L.,
Song
G.,
Selections of appropriate regimen of high-dose chemotherapy combined with adoptive cellular therapy with dendritic and cytokine-induced killer cells improved progression-free and overall survival in patients with metastatic breast cancer: reargument of such contentious therapeutic preferences. Clinical & translational oncology : official publication of the Federation of Spanish Oncology Societies and of the National Cancer Institute of Mexico.
2013;
15
:
780-8
.
View Article Google Scholar -
Zhang
Y.,
Wang
S.,
Yang
B.,
Lu
S.,
Du
Y.,
Liu
H.,
Adjuvant treatment for triple-negative breast cancer: a retrospective study of immunotherapy with autologous cytokine-induced killer cells in 294 patients. Cancer Biology & Medicine.
2019;
16
(2)
:
350-60
.
View Article PubMed Google Scholar -
M. Li,
Y. Wang,
F. Wei,
X. An,
N. Zhang,
S. Cao,
B. Ren,
X. Zhang,
X. Ren,
Efficiency of cytokine-induced killer cells in combination with chemotherapy for triple-negative breast cancer. .
. Journal of breast cancer.
2018;
21
(2)
:
150-7
.
View Article Google Scholar -
K. Pan,
X.X. Guan,
Y.Q. Li,
J.J. Zhao,
J.J. Li,
H.J. Qiu,
D.S. Weng,
Q.J. Wang,
Q. Liu,
L.X. Huang,
J. He,
Clinical Activity of Adjuvant Cytokine-Induced Killer Cell Immunotherapy in Patients with Post-Mastectomy Triple-Negative Breast Cancer. Clinical Cancer Research.
2014;
20
(11)
:
3003-11
.
View Article PubMed Google Scholar -
YJ
Zhao,
N
Jiang,
QK
Song,
JP
Wu,
YG
Song,
HM
Zhang,
F
Chen,
L
Zhou,
XL
Wang,
XN
Zhou,
HB
Yang,
Continuous DC-CIK infusions restore CD8+ cellular immunity, physical activity and improve clinical efficacy in advanced cancer patients unresponsive to conventional treatments. Asian Pacific Journal of Cancer Prevention.
2015;
16
(6)
:
2419-23
.
View Article Google Scholar -
Wang
X.,
Ren
J.,
Zhang
J.,
Prospective study of cyclophosphamide, thiotepa, carboplatin combined with adoptive DC-CIK followed by metronomic cyclophosphamide therapy as salvage treatment for triple negative metastatic breast cancers patients (aged <45). Clinical & translational oncology : official publication of the Federation of Spanish Oncology Societies and of the National Cancer Institute of Mexico.
2016;
18
:
82-7
.
View Article Google Scholar -
Q
Mao,
L
Li,
C
Zhang,
Y
Sun,
S
Liu,
S
Cui,
Clinical effects of immunotherapy of DC-CIK combined with chemotherapy in treating patients with metastatic breast cancer. Pakistan journal of pharmaceutical sciences.
2015;
28
(Supplement)
:
1055-58
.
-
Lin
M.,
Liang
S.,
Jiang
F.,
Xu
J.,
Zhu
W.,
Qian
W.,
2003-2013, a valuable study: autologous tumor lysate-pulsed dendritic cell immunotherapy with cytokine-induced killer cells improves survival in stage IV breast cancer. Immunology Letters.
2017;
183
:
37-43
.
View Article PubMed Google Scholar -
Golay
J.,
Martinelli
S.,
Alzani
R.,
Cribioli
S.,
Albanese
C.,
Gotti
E.,
Cord blood-derived cytokine-induced killer cells combined with blinatumomab as a therapeutic strategy for CD19+ tumors. Cytotherapy.
2018;
20
(8)
:
1077-88
.
View Article PubMed Google Scholar -
Czerniecki
B.J.,
Koski
G.K.,
Koldovsky
U.,
Xu
S.,
Cohen
P.A.,
Mick
R.,
Targeting HER-2/neu in early breast cancer development using dendritic cells with staged interleukin-12 burst secretion. Cancer Research.
2007;
67
(4)
:
1842-52
.
View Article PubMed Google Scholar -
Peoples
G.E.,
Gurney
J.M.,
Hueman
M.T.,
Woll
M.M.,
Ryan
G.B.,
Storrer
C.E.,
Clinical trial results of a HER2/neu (E75) vaccine to prevent recurrence in high-risk breast cancer patients. Journal of Clinical Oncology.
2005;
23
(30)
:
7536-45
.
View Article PubMed Google Scholar -
Svane
I.M.,
Pedersen
A.E.,
Johnsen
H.E.,
Nielsen
D.,
Kamby
C.,
Gaarsdal
E.,
Vaccination with p53-peptide-pulsed dendritic cells, of patients with advanced breast cancer: report from a phase I study. Cancer Immunology, Immunotherapy.
2004;
53
(7)
:
633-41
.
View Article PubMed Google Scholar -
Qin
W.,
Xiong
Y.,
Chen
J.,
Huang
Y.,
Liu
T.,
DC-CIK cells derived from ovarian cancer patient menstrual blood activate the TNFR1-ASK1-AIP1 pathway to kill autologous ovarian cancer stem cells. Journal of Cellular and Molecular Medicine.
2018;
22
(7)
:
3364-76
.
View Article PubMed Google Scholar -
Yang
T.,
Zhang
W.,
Wang
L.,
Xiao
C.,
Wang
L.,
Gong
Y.,
Co-culture of dendritic cells and cytokine-induced killer cells effectively suppresses liver cancer stem cell growth by inhibiting pathways in the immune system. BMC Cancer.
2018;
18
(1)
:
984
.
View Article PubMed Google Scholar -
Lan
X.P.,
Chen
Y.G.,
Wang
Z.,
Yuan
C.W.,
Wang
G.G.,
Lu
G.L.,
Immunotherapy of DC-CIK cells enhances the efficacy of chemotherapy for solid cancer: a meta-analysis of randomized controlled trials in Chinese patients. Journal of Zhejiang University. Science. B..
2015;
16
(9)
:
743-56
.
View Article PubMed Google Scholar -
Zhao
Y.,
Qiao
G.,
Wang
X.,
Combination of DC/CIK adoptive T cell immunotherapy with chemotherapy in advanced non-small-cell lung cancer (NSCLC) patients: a prospective patients' preference-based study (PPPS). Clinical & translational oncology : official publication of the Federation of Spanish Oncology Societies and of the National Cancer Institute of Mexico.
2019;
21
:
721-8
.
View Article Google Scholar -
Hu
J.,
Hu
J.,
Liu
X.,
Hu
C.,
Li
M.,
Han
W.,
Effect and safety of cytokine-induced killer (CIK) cell immunotherapy in patients with breast cancer: A meta-analysis. Medicine.
2017;
96
(42)
:
e8310
.
View Article PubMed Google Scholar -
Qiao
G.,
Wang
X.,
Zhou
L.,
Zhou
X.,
Song
Y.,
Wang
S.,
Autologous Dendritic Cell-Cytokine Induced Killer Cell Immunotherapy Combined with S-1 Plus Cisplatin in Patients with Advanced Gastric Cancer: A Prospective Study. Clinical Cancer Research.
2019;
25
(5)
:
1494-504
.
View Article PubMed Google Scholar -
Wang
Z.X.,
Cao
J.X.,
Wang
M.,
Li
D.,
Cui
Y.X.,
Zhang
X.Y.,
Adoptive cellular immunotherapy for the treatment of patients with breast cancer: a meta-analysis. Cytotherapy.
2014;
16
(7)
:
934-45
.
View Article PubMed Google Scholar -
Gammaitoni
L.,
Giraudo
L.,
Macagno
M.,
Leuci
V.,
Mesiano
G.,
Rotolo
R.,
Cytokine-Induced Killer Cells Kill Chemo-surviving Melanoma Cancer Stem Cells. Clinical Cancer Research.
2017;
23
(9)
:
2277-88
.
View Article PubMed Google Scholar -
Gammaitoni
L.,
Giraudo
L.,
Leuci
V.,
Todorovic
M.,
Mesiano
G.,
Picciotto
F.,
Effective activity of cytokine-induced killer cells against autologous metastatic melanoma including cells with stemness features. Clinical Cancer Research.
2013;
19
(16)
:
4347-58
.
View Article PubMed Google Scholar -
Mesiano
G.,
Grignani
G.,
Fiorino
E.,
Cytokine Induced Killer cells are effective against sarcoma cancer stem cells spared by chemotherapy and target therapy. Oncoimmunology.
2018;
7
:
e1465161-e
.
View Article Google Scholar -
Magnani
C.F.,
Gaipa
G.,
Belotti
D.,
Matera
G.,
Tettamanti
S.,
Cabiati
B.,
Donor-Derived CD19 CAR Cytokine Induced Killer (CIK) Cells Engineered with Sleeping Beauty Transposon for Relapsed B-Cell Acute Lymphoblastic Leukemia (B-ALL). Blood.
2019;
134
:
200
.
View Article Google Scholar -
Hombach
A.A.,
Rappl
G.,
Abken
H.,
Arming cytokine-induced killer cells with chimeric antigen receptors: CD28 outperforms combined CD28-OX40 "super-stimulation". Molecular therapy : the journal of the American Society of Gene Therapy.
2013;
21
:
2268-77
.
View Article Google Scholar -
Ren
X.,
Ma
W.,
Lu
H.,
Yuan
L.,
An
L.,
Wang
X.,
Modification of cytokine-induced killer cells with chimeric antigen receptors (CARs) enhances antitumor immunity to epidermal growth factor receptor (EGFR)-positive malignancies. Cancer Immunology, Immunotherapy.
2015;
64
(12)
:
1517-29
.
View Article PubMed Google Scholar -
Chen
D.,
Sha
H.,
Hu
T.,
Dong
S.,
Zhang
J.,
Liu
S.,
Cytokine-induced killer cells as a feasible adoptive immunotherapy for the treatment of lung cancer. Cell Death & Disease.
2018;
9
(3)
:
366
.
View Article PubMed Google Scholar -
Verneris
M.R.,
Ito
M.,
Baker
J.,
Arshi
A.,
Negrin
R.S.,
Shizuru
J.A.,
Engineering hematopoietic grafts: purified allogeneic hematopoietic stem cells plus expanded CD8+ NK-T cells in the treatment of lymphoma. Biology of blood and marrow transplantation : journal of the American Society for Blood and Marrow Transplantation.
2001;
7
:
532-42
.
View Article Google Scholar -
Tang
Q.,
Grzywacz
B.,
Wang
H.,
Kataria
N.,
Cao
Q.,
Wagner
J.E.,
Blazar
B.R.,
Miller
J.S.,
Verneris
M.R.,
Adoptive immunotherapy with cytokine-induced killer cells for patients with relapsed hematologic malignancies after allogeneic hematopoietic cell transplantation. Biology of blood and marrow transplantation : journal of the American Society for Blood and Marrow Transplantation.
2008;
17
:
1679-87
.
View Article Google Scholar -
Laport
G.G.,
Sheehan
K.,
Baker
J.,
The anti-tumour activity of allogeneic cytokine-induced killer cells in patients who relapse after allogeneic transplant for haematological malignancies. Bone Marrow Transplantation.
2011;
47
:
957-66
.
View Article Google Scholar -
Linn
Y.C.,
Niam
M.,
Chu
S.,
Choong
A.,
Yong
H.X.,
Heng
K.K.,
The anti-tumour activity of allogeneic cytokine-induced killer cells in patients who relapse after allogeneic transplant for haematological malignancies. Bone Marrow Transplantation.
2012;
47
(7)
:
957-66
.
View Article PubMed Google Scholar -
Introna
M.,
Borleri
G.,
Conti
E.,
Franceschetti
M.,
Barbui
A.M.,
Broady
R.,
Repeated infusions of donor-derived cytokine-induced killer cells in patients relapsing after allogeneic stem cell transplantation: a phase I study. Haematologica.
2007;
92
(7)
:
952-9
.
View Article PubMed Google Scholar -
Introna
M.,
Lussana
F.,
Algarotti
A.,
Gotti
E.,
Valgardsdottir
R.,
Micò
C.,
Phase II Study of Sequential Infusion of Donor Lymphocyte Infusion and Cytokine-Induced Killer Cells for Patients Relapsed after Allogeneic Hematopoietic Stem Cell Transplantation. Biology of Blood and Marrow Transplantation.
2017;
23
(12)
:
2070-8
.
View Article PubMed Google Scholar -
Introna
M.,
Franceschetti
M.,
Ciocca
A.,
Borleri
G.,
Conti
E.,
Golay
J.,
Rapid and massive expansion of cord blood-derived cytokine-induced killer cells: an innovative proposal for the treatment of leukemia relapse after cord blood transplantation. Bone Marrow Transplantation.
2006;
38
(9)
:
621-7
.
View Article PubMed Google Scholar -
Zhang
Z.,
Zhao
X.,
Zhang
T.,
Wang
L.,
Yang
L.,
Huang
L.,
Phenotypic characterization and anti-tumor effects of cytokine-induced killer cells derived from cord blood. Cytotherapy.
2015;
17
(1)
:
86-97
.
View Article PubMed Google Scholar -
Zhang
Z.,
Wang
L.,
Luo
Z.,
Zhao
X.,
Huang
J.,
Li
H.,
Efficacy and safety of cord blood-derived cytokine-induced killer cells in treatment of patients with malignancies. Cytotherapy.
2015;
17
(8)
:
1130-8
.
View Article PubMed Google Scholar -
Wang
L.,
Huang
S.,
Dang
Y.,
Li
M.,
Bai
W.,
Zhong
Z.,
Cord blood-derived cytokine-induced killer cellular therapy plus radiation therapy for esophageal cancer: a case report. Medicine.
2014;
93
(28)
:
e340
.
View Article PubMed Google Scholar -
Castiglia
S.,
Adamini
A.,
Rustichelli
D.,
Castello
L.,
Mareschi
K.,
Pinnetta
G.,
Cytokines induced killer cells produced in good manufacturing practices conditions: identification of the most advantageous and safest expansion method in terms of viability, cellular growth and identity. Journal of Translational Medicine.
2018;
16
(1)
:
237
.
View Article PubMed Google Scholar -
Palmerini
P.,
Dalla Pietà
A.,
Sommaggio
R.,
Ventura
A.,
Astori
G.,
Chieregato
K.,
A serum-free protocol for the ex vivo expansion of Cytokine-Induced Killer cells using gas-permeable static culture flasks. Cytotherapy.
2020;
22
(9)
:
511-8
.
View Article PubMed Google Scholar -
Mareschi
K.,
Adamini
A.,
Castiglia
S.,
Rustichelli
D.,
Castello
L.,
Mandese
A.,
Cytokine-Induced Killer (CIK) Cells, In Vitro Expanded under Good Manufacturing Process (GMP) Conditions, Remain Stable over Time after Cryopreservation. Pharmaceuticals (Basel, Switzerland).
2020;
13
(5)
:
93
.
View Article PubMed Google Scholar -
Liang
X.,
Hu
X.,
Hu
Y.,
Zeng
W.,
Zeng
G.,
Ren
Y.,
Recovery and functionality of cryopreserved peripheral blood mononuclear cells using five different xeno-free cryoprotective solutions. Cryobiology.
2019;
86
:
25-32
.
View Article PubMed Google Scholar
Comments
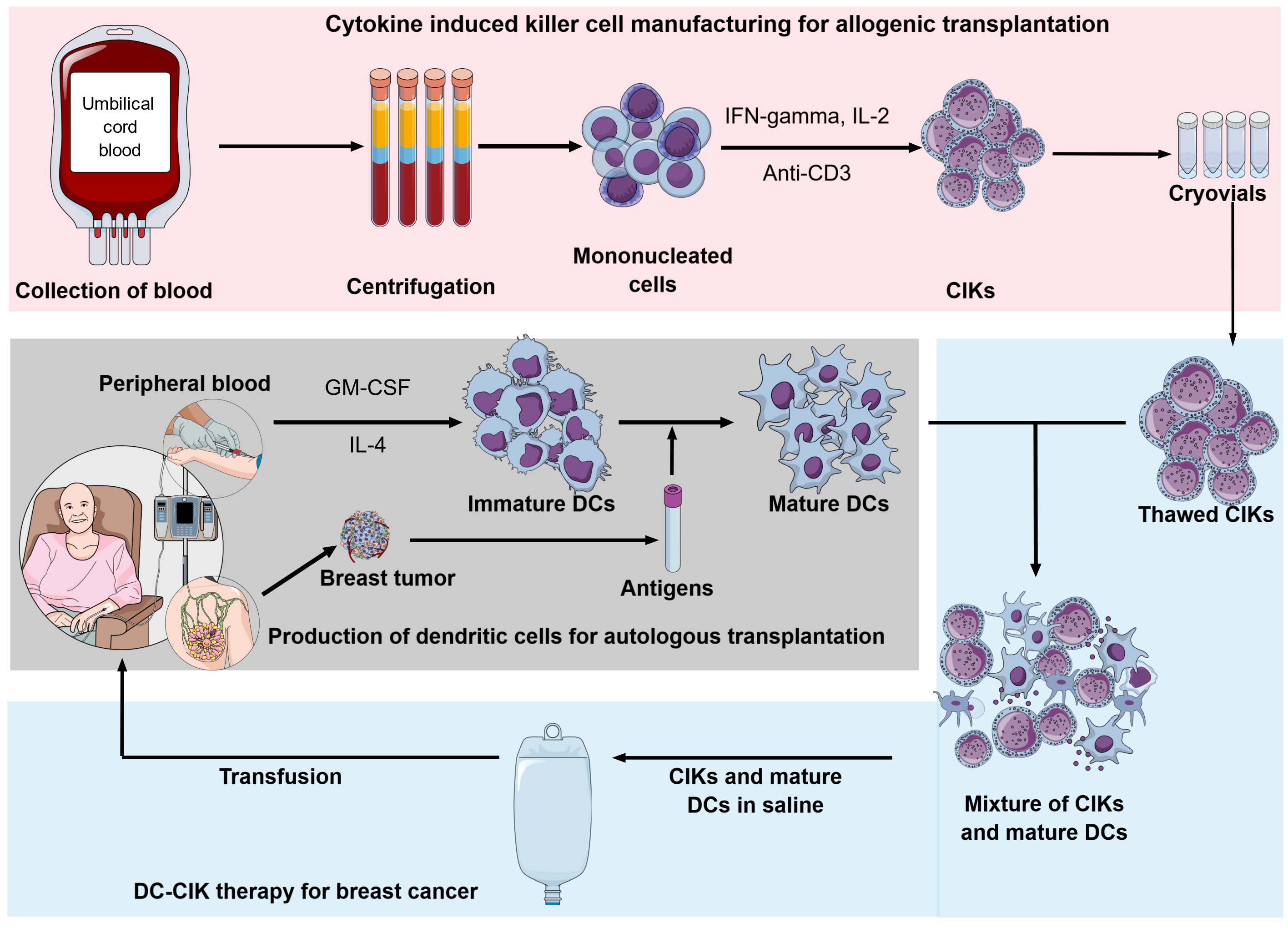
Article Details
Volume & Issue : Vol 8 No 11 (2021)
Page No.: 4700-4717
Published on: 2021-11-30
Citations
Copyrights & License

This work is licensed under a Creative Commons Attribution 4.0 International License.
Search Panel
- HTML viewed - 8821 times
- PDF downloaded - 3943 times
- XML downloaded - 0 times