Abstract
Coronavirus disease 2019 (COVID-19) has caused nearly 15 million deaths worldwide. The rapid development of COVID-19 vaccines and anti-viral drugs significantly decreased the level of mortality related to COVID-19. However, post-COVID-19 pulmonary fibrosis has become a severe problem for some COVID-19 patients. The previous articles present the results of mesenchymal stem cell (MSC) transplantation to treat COVID-19 patients; in this article, we would like to discuss the potential of MSC transplantation to treat and improve post-COVID-19 pulmonary fibrosis. MSCs exhibit immune modulation and anti-inflammation that can control the inflammation caused by coronavirus 2 infection and the cytokine storm that some patients experience during COVID-19. The anti-fibrotic qualities of MSCs have also been demonstrated both in vitro and in vivo. Based on the current information about the anti-fibrotic effects of MSCs, MSC transplantation can be used to improve post-COVID-19 pulmonary fibrosis.
Introduction
The coronavirus disease 2019 (COVID-19) pandemic is the fifth documented pandemic since the 1918 flu pandemic. The first reported case of COVID-19 was recorded in Wuhan, China, before the virus spread worldwide. Since 2019, there have been nearly 15 million deaths due to COVID-19. Vaccines and anti-viral drugs significantly decreased mortality related to COVID-19 around the world. However, the rate of COVID-19 infections has continuously increased in most countries, even after vaccination. Some reports have shown that although the mortality was reduced, the rates of post-COVID-19 syndrome and post-COVID-19 pulmonary fibrosis (PF) were not significantly reduced.
Post-COVID-19 PF has mainly been observed in patients with comorbidities such as diabetes, hypertension, or coronary disease1. In 2020, Wu et al. showed that up to 40% of patients who had recovered from COVID-19 may develop ARDS; 20% of them may develop PF2. In some other reports, PF is estimated to affect about 1/3 of the patients hospitalized with SARS-CoV-23, 4.
Therefore, post-COVID-19 PF poses a serious threat to patients who have recovered from COVID-19. Many of the potential treatments reported in the literature can improve post-COVID-19 PF; however, there is no fully proven treatment for this condition. This review aims to comprehensively review PF in post-COVID-19 patient, some current treatments for PF, and some early results using mesenchymal stem cells (MSCs) for post-COVID-19 PF treatment.
SARS-CoV-2 and COVID-19 Pathophysiology
Virus SARS-CoV-2 profile and viral life cycle
Severe acute respiratory syndrome coronavirus 2 (SARS-CoV-2), which is responsible for the COVID-19 pandemic, belongs to the family Coronaviridae. The Coronaviridae family has 2 subfamilies, Coronavirinae and Torovirinae. Coronavirinae includes seven coronavirus-caused respiratory diseases in human, which are divided into two groups: normal coronaviruses (HCoV-229E, HCoV-NL63, HCoV-HKU1, HCoV-OC43) and novel coronaviruses (MERS-CoV, SARS-CoV and SARS-CoV-2)5. The normal coronaviruses usually causes acute mild upper tract respiratory diseases (except NL63), while the novel coronaviruses can lead to pneumonia and severe acute respiratory syndrome (SARS). Among the three novel coronaviruses, SARS-CoV-2 has had the lowest case fatality rate (CFR)—under 5% — but the highest level of transmissibility.
Coronaviruses (CoVs) have large single-stranded positive-sense RNA genomes—around 30 kilobases, containing a 5’-cap and 3’ poly (A) tail structure6. CoVs have a lipid bilayer membrane and include four main structural proteins: nucleocapsid (N) protein, membrane (M) protein, envelope (E) protein and, most importantly, spike (S) protein6, 7. S protein plays a pivotal role in the initial attachment and penetration of the virus to the host cell by binding to the angiotensin-converting enzyme 2 receptor (ACE-2). The spike protein is composed of two subunits, S1 and S2. S1 mediates the binding of the virus to the host cell receptor, while S2 is responsible for the fusion of virion and cell membrane7. Inside the host cell, the virus replicates and performs exocytosis, after which it is ready to invade adjacent cells.
COVID-19 pathophysiology
ACE-2 has been determined to be a main functional receptor for SARS-CoV, HCoV-NL63, and SARS-CoV-2. ACE-2 has primarily been expressed in type II pulmonary epithelial cells, so the respiratory system is the easiest method for viruses to infect patients. However, ACE-2 has also been observed in nasal goblet cells, gastrointestinal epithelial cells, pancreatic β cells, and renal podocytes6, 8, leading to digestive issues, myocarditis, kidney diseases and, ultimately, multiple organ dysfunction syndromes. Compared to SARS-CoV, SARS-CoV-2 has a 10–20 times higher affinity for ACE-2 receptors9, which is why there have been more COVID-19-related myocarditis cases (even among those who are young and have no comorbidities) than in the 2002-2004 SARS outbreak in Hong Kong.
The renin-angiotensin system (RAS) plays an essential role in regulating blood pressure and homeostasis. In the lungs, angiotensin I (Ang I) metabolizes to angiotensin II (Ang II) by the ACE. Ang II acts through angiotensin type I and type II receptors (AT1R and AT2R), leading to the constriction of vascular, water, and sodium retention, stimulation of chronic inflammation, fibrosis, and lung damage. Conversely, the ACE-2 converts Ang II into Ang 1-7, which exerts vasodilation through binding Mas receptor, thus inhibiting inflammation and preventing pulmonary diseases and edema10. Therefore, the ACE2/Ang1-7/Mas axis is antagonized to the ACE/AngII/AT1R pathway (classic RAS). When SARS-CoV-2 binds to cells with a high affinity, the bond between the virus and ACE-2 is formed, leading to the down regulation of the ACE-2 axis. This imbalance is partly responsible for the acute and chronic inflammation (due to overactivity of RAS), resulting in medium to severe COVID-19 symptoms and long-term complications.
Many researchers have concluded that there is a correlation between the increasing level of pro-inflammatory cytokines and the outcome of COVID-19 patients; cytokine storm syndrome is the main factor that causes severe and fatal complications in patients11, 12, 13, 14. Cytokines are small protein molecules secreted through the interaction between cells, including pro-inflammatory and anti-inflammatory cytokines15. The virus invades the nasal epithelial cell, replicates, and immigrates to the upper and lower respiratory cells, then to lymphocytes. With COVID-19, cytokines are produced not only through PAMPs (pathogen-associated molecular pattern molecules) and DAMPs (damage-associated molecular pattern molecules) pathways but also during the crosstalk between epithelial cells and immune cells via the trigger of macrophages. In mild to moderate cases of COVID-19, interferon-1 (IFN-1, which includes IFN-α and IFN-ß) is efficiently and productively secreted, functioning as a lung protector. Otherwise, the inflammation is regulated by the balancing between pro-inflammatory (TNF-α, IL-1, IL-6) and anti-inflammatory (IL-10, IL-1) cytokines. This facilitates quicker and more effective virus clearance without any serious complications.
On the other hand, in patients with severe to critical disease, there is not only impaired INF-1 production and activity accelerating DAMPs and PAMPs pathways but also exacerbated inflammation, with an excess of pro-inflammatory cytokines (TNF-α, IL-6), leading to the cytokines auto-amplification and cytokine storm, resulting in ARDS, multi-organ failure or even death12, 14. Moreover, the imbalance of the ACE and ACE-2 axis that leads to the ACE/AngII/AT1R over-activity will exaggerate the releasing soluble TNF-α, HB-EGF, and IL-6Rα to adjacent cells and activate NK-kB and STAT3 to produce more IL-68. Serum IL-6 and TNF-α levels can be used to reliably and independently predict poorer prognoses16. Therefore, ACE/AngII blockers and anti-IL-6 have recently been considered viable medication for treating disease of medium to critical severity.
Immune response to SARS-CoV-2 and COVID-19
Besides local immune activity (innate cell immunopathology and plasma cytokines signature), understanding the specific immune response is a vital part of controlling the virus invasion, with three major components: antibodies (produced by B cells), CD4+ T cells, and CD8+ T cells. The adaptive immune response works slower than innate immunity; it takes time to proliferate and differentiate naive cells into effector cells. When the virions, ingested by antigen-presenting cells (APC), are recognized by CD4+ T cells, the T helper cells activate both cytotoxic T cells to destroy infected cells and virus, and B cells produce antibodies. If the adaptive immune response works fast and effectively, eliminating the circulating virions, the disease can be controlled; patients usually have no symptoms. Conversely, T cells and antibodies may not be activated soon enough, in which case the local immune activity can lead to over-activity in cytokine production. In the case of SARS-CoV-2 and COVID-19, the virus can avoid or delay innate immunity related to INF-1 efficiently, leading to the overproduction of pro-inflammatory cytokines17, 18, 19, 20.
Post-COVID-19 syndrome/COVID-19 long-term complications and COVID-19 pulmonary fibrosis
Although the accurate pathological mechanism of long-term COVID-19 complications is still a matter of controversy, several mechanisms have been reported to be associated with the following disease sequelae: (1) direct viral tissue damages due to the diverse locations of ACE-2 expression in epithelial cells leads to multiorgan injuries; (2) the high expression of ACE-2 in endothelial cells affects the complexity of blood vessels, which may lead to coagulation and thrombosis; (3) the consequences of cytokine storm syndrome; (4) the imbalance of ACE axis and ACE-2 axis, leading to vascular constriction, lung fibrosis, and chronic inflammation; (5) immune system dysregulation (has been proven by finding auto-reactive T cells in patients with fatal cases of COVID-19, similar to autoimmune disease patients)8, 14, 21, 22.
Recent research has shown that COVID-19 patients with prolonged symptoms can have SARS-CoV-2 detection blood tests that are either (1) PCR-negative or (2) low-level trace PCR-positive. Patients with trace PCR-positive results may experience long-lasting symptoms, even over 3 months after the initial infection. Remarkably, in long-lasting symptomatic patients who are trace PCR-positive, SARS-CoV-2-specific CD8+ T cells increase in breadth and magnitude, which may be related to the mechanism by which long-term complications occur19, 22, 23, 24.
Many systems are affected by long-term COVID-19, including: respiratory (dyspnea, pulmonary fibrosis, lung function abnormalities, ventilator/oxygen dependence, etc.), cardiological (chest pain, arrhythmia, ventricular systolic dysfunction, heart failure, etc.), hematological (thrombosis, hypoxia, etc.), neurological (fatigue, myalgia, headache, migraine, insomnia, etc.), dermatological (urticaria, alopecia, rash, etc.), urological (acute kidney injury, renal dysfunctional, etc.), digestive (nausea, vomiting, poor appetite, etc.) and so on8. In this review, we focused on post-COVID-19 lung fibrosis.
Pulmonary fibrosis is also called interstitial lung disease or diffuse parenchymal lung disease. The term “post-COVID19 fibrosis” includes any “fibrotic-like” conditions (reversible diseases) and fibrosis (irreversible diseases) with specific CT imaging features25. The high level of transforming growth factor-beta 1 (TGF-β1) is considered to be the main factor for organizing pneumonia (based on recent knowledge of past influenza and SARS pandemics). The mechanism is the production of profibrotic agents such as TGF-β1, PDGF, VEGF, EGF, etc., which leads to the activation of fibroblasts (which stay resident and recruit from the blood) and subsequent differentiation into myofibroblasts, stimulating collagen synthesis26. The fibroblasts and myofibroblasts will organize into fibrotic foci. The growth factors targeting tyrosine kinase pathways are released continuously to promote fibrotic foci formation and evolution in a fibrosed lung. Collagen type 3 is a predominant form of collagen during the early stage, while collagen type 1 is revealed during the late stage.
In SARS-CoV-2 infection, the exact mechanism of fibrosis is still unknown; however, it is believed to be multifactorial, primarily a result of the release of cytokines and abnormal coagulopathy (which is the difference between COVID-19-induced ARDS and classic ARDS), as well as the down regulation of the ACE-2 axis (as described above). In addition to these are two iatrogenic factors, namely, oxygenic toxicity and ventilator-induced lung injury. Patients exposed to high doses of oxygen experience enhanced levels of oxygen-derived free radicals, which damage the epithelial cells. Patients with severe disease who are supported by prolonged mechanical ventilation experience extended high pressure inside their stiff lungs; this physical stress also contributes to pulmonary fibrosis26.
The clinical symptoms of organizing pulmonary fibrosis differ from patient to patient; however, all are very similar to those common of respiratory diseases, including prolonged dry cough, shortness of breath, fatigue, decreased exercise tolerance, or even a lack of symptoms. Risk factors such as age, comorbidities (hypertension, diabetes, cardiovascular disease, cancer, pulmonary tuberculosis, etc.), prolonged ICU stay and ventilation period, smoking, and a history of alcohol abuse play an important role in the progression of severe COVID-1927. Specific paraclinical test features consist of the reduction of lung diffusion capacity of carbon monoxide (DLCO) and the formations associated with lung scarring, which are identified via CT scan, such as traction dilation of bronchi, honeycombing, pleural thickening or bands in the focal area28. According to Wu et al., 30% of COVID-19 patients suffer from at least some lung complication at 9 months after hospitalization; a third of them develop fibrotic-like damages without any reduction trends29. Another study by Wu et al. shows a similar result: 40% of COVID-19 patients develop ARDS and 20% of ARDS cases are severe, with imaging results showing diffuse alveolar damage; about 10% of recovered patients experience lung fibrosis30. Long-term research carried out on SARS patients for 15 years has shown that the lesions due to lung damage can be reduced within 12 months after recovery, without any evolution or changes observed 14 years later31; post-COVID-19 lesions due to lung damage are now considered to progress in a similar way.
Current status of post-COVID-19 pulmonary fibrosis treatment
Currently, there are no fully proven treatment methods for COVID-induced pulmonary fibrosis. Different approaches are being researched, with a focus on anti-inflammatory and anti-fibrotic drugs. Nintedanib and pirfenidone—oral therapeutics commonly used to treat idiopathic pulmonary fibrosis (IPF)—are the most commonly investigated anti-fibrotic medicines. In IPF patients, nintedanib and pirfenidone have been shown to inhibit the reduction of lung function and increase life expectancy32. Nintedanib (6-methoxycarbonyl-substituted indolinone) prevents pro-fibrotic signaling pathways through mediators (TGF-β1, PDGF, VEGF, EGF). Pirfenidone (5-methyl-1-phenyl-2-(1H)-pyridone) works via multiple mechanisms by regulating pro-fibrotic and pro-inflammatory agents, inhibiting the activation of collagen synthesis and fibroblast differentiation through TGF-β1 and playing an antioxidant role. Despite their potential, the side effects and drug interactions must be taken into account. Several common reactions, such as diarrhea, fatigue, and poor appetite, are similar to COVID-19 symptoms, which can affect physicians’ evaluations of patients. Nintedanib and pirfenidone can also cause damage to the liver, which can be seriously affected by COVID-19 itself28. When using anticoagulants, it is necessary to delay Nintedanib, which can increase the risk of bleeding33.
Besides, several new medicines have been the subject of ongoing clinical trials around the world since 2020. These include two multicenter, randomized, placebo-controlled research projects with Treamid (NCT04527354), Bov Hyaluronidase azoximer (NCT04645368), and LYT-100 (NCT04652518), one multicenter, randomized trial compared to standard therapy with Anluohuaxian (NCT0433426) and many single-center, randomized, placebo-controlled trials with Collagen Polyvinylpyrrolidone, Prednisone, Genistein, Tetrandrine, etc. In addition, cell therapy—especially using mesenchymal stem cells—has been reported as a potential treatment method.
Mesenchymal stem cells and their bioeffects targeting pulmonary fibrosis
Preclinical trials and clinical trials for pulmonary fibrosis
To date, there have been more than 20 reports on the use of MSCs for PF in animal models. Most of the studies used MSCs from bone marrow or adipose tissues. Other kinds of MSCs used to treat PF in animal models include umbilical cord MSCs, amniotic membrane MSCs, human embryonic MSCs, and human menstrual blood–derived MSCs. In a 2021 review by Li et al.34, 12 animal studies showed that animals treated with MSCs had significantly improved survival rates compared to the control group (p < 0.001)34. Moreover, in this meta-analysis review, Li et al. (2021) showed that MSC transplantation clearly reduced pulmonary fibrosis scores compared to the control group (p < 0.01)34.
In a 2013 study, Tzouvelekis et al. used adipose-derived stromal vascular fraction (SVF) to treat 14 PF patients35. In this study, the researchers used a low dose of SVF (0.5 million cells per kg of body weight per infusion). Although no cases of serious adverse events were reported, treatment efficacy was not significantly improved in patients who received transplants35.
Averyanov et al. (2019) used a high dose of MSCs to treat PF in 20 human patients. This clinical trial showed that the cumulative dose of 2 billion cells is safe and tolerable in PF patients. After 52 weeks of follow-up, the authors confirmed that MSC transplantation with a cumulative high dose is effective for treating rapidly progressing PF36.
A study by Campo et al. (2021) transplanted autologous bone marrow MSCs (BM-MSCs) for 13 patients37. The transplantation of BM-MSCs was determined to be safe, and the mean forced vital capacity declined by 8.1% at 3 months37.
ClinicalTrials.gov Identifier | Title | Phase/Patients | Kind of cells | Methods | Country |
---|---|---|---|---|---|
NCT04909892 | Study of Allogeneic Adipose-Derived Mesenchymal Stem Cells to Treat Post COVID-19 "Long Haul" Pulmonary Compromise | Phase II (60 patients) | Allogeneic culture-expanded adipose-derived mesenchymal stem cells | MSCs will be administered intravenously on Day 0, Day 2, and Day 4 with 2 groups of 2 doses: one vial, ~18.5 million cells per infusion and ~37 million cells per infusion | USA |
NCT05126563 | Randomized Double-Blind Phase 2 Study of Allogeneic HB-adMSCs for the Treatment of Chronic Post-COVID-19 Syndrome (HBPCOVID02) | Phase II (80 patients) | Allogeneic culture-expanded adipose-derived mesenchymal stem cells | Dose: 200 million Route: Intravenous Regimen: Weeks 0, 2, 6, and 10. | USA |
NCT04798066 | HBPCOV01:"Intermediate Size Expanded Access Protocol for the Treatment of Post-COVID-19 Syndrome" | Phase I (5 patients) | Autologous adipose derived mesenchymal stem cells | Dose: 200 million HB-adMSCs Route: intravenous infusion only, Regimen: a treatment duration of 14 weeks | USA |
NCT05116761 | ExoFlo™ Infusion for Post-Acute COVID-19 and Chronic Post-COVID-19 Syndrome | Phase I/II (60 patients) | Bone Marrow Mesenchymal Stem Cell Derived Extracellular Vesicles | Dose: Normal saline 85 mL and ExoFlo 15 mL, which is 10.5 x 10 8 EV Route: Intravenous | USA |
Mechanisms of MSCs for pulmonary fibrosis
Effects of MSCs on COVID-19 treatment, including (1) anti-viral effects, (2) immune modulation, and (3) lung regeration, have been comprehensively discussed in some previous publications38, 39. Unlike COVID-19 treatment, post-COVID-19 treatment can be effective if the fibrosis process is reversed. Ideally, the treatment for fibrosis should achieve three steps of fibrotic tissue regeneration, namely, the inhibition or reduction in fibrosis progression (via inhibiting inflammation, reducing myofibroblast activity, and preventing further collagen synthesis), the degradation of the accumulated/existing collagen, and the triggering of lung regeneration (Figure 1). MSCs can target three therapeutic mechanisms of fibrotic lung regeneration.
MSCs can display immune modulation that significantly reduces inflammation by suppressing T lymphocytes, B lymphocytes, dendritic cells, and NK cells and stimulating regulatory T cells. MSCs also secrete an array of anti-inflammatory cytokines (reviewed in Pham and Vu, 202040). In a model of radiation-induced pulmonary fibrosis in animals, Dong et al. (2015) showed that MSCs can reduce fibrosis in PF in animals by stimulating the endogenous secretion of HGF and PGE241. MSCs also secrete HGF that inhibits epithelial-to-mesenchymal cell transition and promotes myofibroblast apoptosis42. The role of MSC-derived HGF in protection from bleomycin-induced PF was confirmed by Cahill et al. (2016). Cahill et al. (2016) compared the effects of MSCs and HGF-knockdown MSCs on bleomycin-induced PF and showed that HGF knockdown MSCs could not protect against fibrosis in vivo43.
The degradation of existing cumulated collagen in fibrotic lungs is an essential step in fibrotic lung regeneration. Transplantation of MSCs can activate the host’s macrophages, especially M2 macrophage, to synthesize the MMP-9 degrading the collagen44. Indeed, in animal models of PF, UCMSC transplantation reduced collagen deposition (determined by Sirius red staining)44. Indeed, overexpression of MMP9 in macrophages can attenuate bleomycin-induced PF45. In vitro, Khan et al. (2017) also showed that MSCs could decrease Col1A1 deposition by increasing the ratio of MMP-1 to TIMP-146.
The last step of anti-fibrosis in PF is tissue regeneration. Previous studies have confirmed that MSCs could produce and secrete some growth factors that can activate the local stem cells for tissue homeostasis47, 48. Chu et al. (2020) determined the indirect way that MSC transplantation triggers tissue regeneration in PF through TLR-444. Transplanted MSCs will promote the expression of TLR-4 in the host’s alveolar epithelial cells. TLR-4 promotes alveolar progenitor cell renewal and prevents severe pulmonary fibrosis49.
Xiao et al. (2020) reported that MSC-derived exosomes could reverse the progression of LPS-induced lung injury and fibrosis50. Exosomes from MSCs transmitted miR-23a-3p and miR-182-5p to the MLE-12 cells and inhibited the NF-kB and Hedgehog pathways by silencing lkbkb and destabilizing IKKbeta50. Zhang et al. (2021) also demonstrated that exosomes from BMMSCs can reverse the epithelial-mesenchymal transition to alleviate silica-induced pulmonary fibrosis51. Indeed, exosomes from BMMSCs caused an increase in epithelial markers (including E-cadherin and cytokeratin 19) and reduced the expression of fibrosis marker proteins (including alpha-SMA). The authors proposed that a mechanism for the anti-fibrotic effects of exosomes could be the attenuation of the Wnt/beta-catenin signaling pathway51.
An update on usage of mesenchymal stem cells for post-COVID-19
Rahulan et al. (2022) recently used MSC transplantation to treat five patients with post-COVID ARDS on ECMO who were referred for lung transplantation52. All patients received 5 million cells per kilogram infused over 30 minutes for 3 doses on days 0, 3, and 6. The results showed that all patients tolerated MSCs well without experiencing any observable side effects. Three patients recovered from post-COVID-19 and weaned off ECMO successfully; 1 patient did not improve and expired; 1 patient did not recover and underwent lung transplantation52.
On the clinicaltrials.gov website, there are three active clinical trials using mesenchymal stem cells for post-COVID-19 treatment and one active clinical trial using extracellular vesicles from MSCs for post-COVID-19 treatment (Table 1). Two clinical trials use allogeneic expanded ADSCs; the clinical trial uses autologous ADSCs. It seems that while umbilical cord-derived MSCs (UCMSCs) are usually used to treat COVID-19 because of the strong immune modulation of UCMSCs, adipose tissue-derived MSCs are used to treat post-COVID-19. Indeed, in the previous publication53, ADSCs were considered a better cell source than BM-MSCs to treat hindlimb ischemia in mice53. In another study, Liu et al. (2020) showed that ADSCs are better than UCMSCs in treating spinal cord injury in animals54. These results show that ADSCs are a more suitable cell source than BMSCs or UCMSCs for tissue regeneration, while UCMSCs are suitable for treating anti-inflammation through their immunomodulation capacity55. However, more studies have confirmed this observation.
In addition to mesenchymal stem cells used to treat post-COVID-19, extracellular vesicles from MSCs are also being used in a clinical trial (NCT05116761). In this study, EVs from BMMSCs were collected and used with a dose of 10.5 x 108 EV per patient.
Conclusion
COVID-19 has become the most serious pandemic on record. Some novel therapeutic treatments and vaccines have significantly reduced mortality; however, post-COVID-19, especially post-COVID-19 pulmonary fibrosis, is on the rise as a new condition affecting many patients who have tested negative for SARS-CoV-2. Although it is difficult to conclude based off of the current evidence that this therapy is efficacious for treating post-COVID-19 PF, the first clinical report of MSC transplantation for post-COVID-19 ARDS on ECMO patients referred for lung transplantation and the current results reported on the usage of MSCs for PF suggest that MSC transplantation should be considered a promising therapy for post-COVID-19. More clinical trials should be performed to evaluate this therapy.
Abbreviations
ACE-2: Angiotensin-converting Enzyme, APC: antigen-presenting cells, COVID-19: Coronavirus disease 2019, MSC: mesenchymal stem cell, PF: pulmonary fibrosis, SARS-CoV-2: Severe acute respiratory syndrome coronavirus 2, SVF: stromal vascular fraction
Acknowledgments
None
Author’s contributions
All authors equally contributed to this work. All authors read and approved the final manuscript.
Funding
None.
Availability of data and materials
Not applicable.
Ethics approval and consent to participate
Not applicable.
Consent for publication
Not applicable.
Competing interests
The authors declare that they have no competing interests.
References
-
Spagnolo
P.,
Balestro
E.,
Aliberti
S.,
Cocconcelli
E.,
Biondini
D.,
Casa
G.D.,
Pulmonary fibrosis secondary to COVID-19: a call to arms?. The Lancet. Respiratory Medicine.
2020;
8
(8)
:
750-2
.
View Article PubMed Google Scholar -
Wu
C.,
Chen
X.,
Cai
Y.,
X. Zhou,
S. Xu,
H. Huang,
L. Zhang,
X. Zhou,
C. Du,
Y. Zhang,
J. Song,
Wu C, Chen X, Cai Y, Zhou X, Xu S, Huang H, Zhang L, Zhou X, Du C, Zhang Y, Song J. Risk factors associated with acute respiratory distress syndrome and death in patients with coronavirus disease 2019 pneumonia in Wuhan, China. JAMA internal medicine.
2020;
180
(7)
:
934-943
.
View Article Google Scholar -
Tale
S.,
Ghosh
S.,
Meitei
S.P.,
Kolli
M.,
Garbhapu
A.K.,
Pudi
S.,
Post-COVID-19 pneumonia pulmonary fibrosis. QJM: An International Journal of Medicine.
2020;
113
(11)
:
837-8
.
View Article PubMed Google Scholar -
Vasarmidi
E.,
Tsitoura
E.,
Spandidos
D.A.,
Tzanakis
N.,
Antoniou
K.M.,
Pulmonary fibrosis in the aftermath of the COVID-19 era (Review). Experimental and Therapeutic Medicine.
2020;
20
(3)
:
2557-60
.
View Article PubMed Google Scholar -
Ludwig
S.,
Zarbock
A.,
Coronaviruses and SARS-CoV-2: A Brief Overview. Anesthesia and Analgesia.
2020;
131
(1)
:
93-6
.
View Article PubMed Google Scholar -
Wang
Y.,
Grunewald
M.,
Perlman
S.,
Coronaviruses: An Updated Overview of Their Replication and Pathogenesis. Methods in Molecular Biology (Clifton, N.J.).
2020;
2203
:
1-29
.
View Article PubMed Google Scholar -
Parasher
A.,
COVID-19: current understanding of its Pathophysiology, Clinical presentation and Treatment. Postgraduate Medical Journal.
2021;
97
(1147)
:
312-20
.
View Article PubMed Google Scholar -
Desai
A.D.,
Lavelle
M.,
Boursiquot
B.C.,
Wan
E.Y.,
Long-term complications of COVID-19. American Journal of Physiology: Cell Physiology.
2022;
322
(1)
:
1-11
.
View Article PubMed Google Scholar -
Shirbhate
E.,
Pandey
J.,
Patel
V.K.,
Kamal
M.,
Jawaid
T.,
Gorain
B.,
Understanding the role of ACE-2 receptor in pathogenesis of COVID-19 disease: a potential approach for therapeutic intervention. Pharmacological Reports.
2021;
73
(6)
:
1539-50
.
View Article PubMed Google Scholar -
Paul
M.,
Poyan Mehr
A.,
Kreutz
R.,
Physiology of local renin-angiotensin systems. Physiological Reviews.
2006;
86
(3)
:
747-803
.
View Article PubMed Google Scholar -
Yang
L.,
Xie
X.,
Tu
Z.,
Fu
J.,
Xu
D.,
Zhou
Y.,
The signal pathways and treatment of cytokine storm in COVID-19. Signal transduction and targeted therapy.
2021;
6
(1)
:
1-20
.
View Article PubMed Google Scholar -
Tang
Y.,
Liu
J.,
Zhang
D.,
Xu
Z.,
Ji
J.,
Wen
C.,
Cytokine Storm in COVID-19: The Current Evidence and Treatment Strategies. Front Immunol.
2020;
11
:
1708
.
View Article PubMed Google Scholar -
Henderson
L.A.,
Canna
S.W.,
Schulert
G.S.,
Volpi
S.,
Lee
P.Y.,
Kernan
K.F.,
On the Alert for Cytokine Storm: immunopathology in COVID-19. Arthritis & Rheumatology (Hoboken, N.J.).
2020;
72
(7)
:
1059-63
.
View Article PubMed Google Scholar -
Mehta
P.,
McAuley
D.F.,
Brown
M.,
Sanchez
E.,
Tattersall
R.S.,
Manson
J.J.,
HLH Across Speciality Collaboration
UK,
COVID-19: consider cytokine storm syndromes and immunosuppression. Lancet.
2020;
395
(10229)
:
1033-4
.
View Article PubMed Google Scholar -
Zhang
J.M.,
An
J.,
Cytokines, inflammation, and pain. International Anesthesiology Clinics.
2007;
45
(2)
:
27-37
.
View Article PubMed Google Scholar -
Valle
D.M. Del,
Kim-Schulze
S.,
Huang
H.-H.,
Beckmann
N.D.,
Nirenberg
S.,
Wang
B.,
An inflammatory cytokine signature predicts COVID-19 severity and survival . Nat Med.
2020;
26
(10)
:
1636-1643
.
View Article PubMed Google Scholar -
Sette
A.,
Crotty
S.,
Adaptive immunity to SARS-CoV-2 and COVID-19. Cell.
2021;
184
(4)
:
861-80
.
View Article PubMed Google Scholar -
Bastard
P.,
Rosen
L.B.,
Zhang
Q.,
Michailidis
E.,
Hoffmann
H.H.,
Zhang
Y.,
Lab
HGID,
Immune Response to COVID Group
NIAID-USUHS,
Clinicians
COVID,
Clinicians
COVID-STORM,
Imagine COVID Group
French COVID Cohort Study Group
Milieu Intérieur Consortium
CoV-Contact Cohort
Amsterdam UMC Covid-19 Biobank
Human Genetic Effort
COVID,
Autoantibodies against type I IFNs in patients with life-threatening COVID-19. Science.
2020;
370
(6515)
.
View Article PubMed Google Scholar -
Blanco-Melo
D.,
Nilsson-Payant
B.E.,
Liu
W.C.,
Uhl
S.,
Hoagland
D.,
M∅ller
R.,
Imbalanced Host Response to SARS-CoV-2 Drives Development of COVID-19. Cell.
2020;
181
(5)
.
View Article PubMed Google Scholar -
Arunachalam
P.S.,
Wimmers
F.,
Mok
C.K.,
Perera
R.A.,
Scott
M.,
Hagan
T.,
Systems biological assessment of immunity to mild versus severe COVID-19 infection in humans. Science.
2020;
369
(6508)
:
1210-20
.
View Article PubMed Google Scholar -
Vabret
N.,
Britton
G.J.,
Gruber
C.,
Hegde
S.,
Kim
J.,
Kuksin
M.,
Immunology of COVID-19: Current State of the Science. Immunity.
2020;
52
(6)
:
910-941
.
View Article PubMed Google Scholar -
Carfì
A.,
Bernabei
R.,
Landi
F.,
Group
Gemelli Against COVID-19 Post-Acute Care Study,
Persistent Symptoms in Patients After Acute COVID-19. Journal of the American Medical Association.
2020;
324
(6)
:
603-5
.
View Article PubMed Google Scholar -
Ngai
J.C.,
Ko
F.W.,
Ng
S.S.,
To
K.W.,
Tong
M.,
Hui
D.S.,
The long-term impact of severe acute respiratory syndrome on pulmonary function, exercise capacity and health status. Respirology (Carlton, Vic.).
2010;
15
(3)
:
543-50
.
View Article PubMed Google Scholar -
Shang
J.,
Ye
G.,
Shi
K.,
Wan
Y.,
Luo
C.,
Aihara
H.,
Structural basis of receptor recognition by SARS-CoV-2. Nature.
2020;
581
(7807)
:
221-224
.
View Article PubMed Google Scholar -
Nabahati
M.,
Ebrahimpour
S.,
Tabari
R. Khaleghnejad,
Mehraeen
R.,
Post-COVID-19 pulmonary fibrosis and its predictive factors: a prospective study. Egyptian Journal of Radiology and Nuclear Medicine.
2021;
52
(1)
:
1-7
.
View Article Google Scholar -
Udwadia
Z.F.,
Koul
P.A.,
Richeldi
L.,
Post-COVID lung fibrosis: the tsunami that will follow the earthquake. Lung India : Official Organ of Indian Chest Society.
2021;
38
(7)
:
41-7
.
View Article PubMed Google Scholar -
Rai
D.K.,
Sharma
P.,
Kumar
R.,
Post covid 19 pulmonary fibrosis. Is it real threat?. Indian journal of tuberculosis.
2021;
68
(3)
:
330-333
.
View Article Google Scholar -
Bazdyrev
E.,
Rusina
P.,
Panova
M.,
Novikov
F.,
Grishagin
I.,
Nebolsin
V.,
Lung Fibrosis after COVID-19: Treatment Prospects. Pharmaceuticals (Basel).
2021;
14
(8)
:
807
.
View Article PubMed Google Scholar -
Wu
X.,
Liu
X.,
Zhou
Y.,
Yu
H.,
Li
R.,
Zhan
Q.,
3-month, 6-month, 9-month, and 12-month respiratory outcomes in patients following COVID-19-related hospitalisation: a prospective study. The Lancet: Respiratory Medicine.
2021;
9
(7)
:
747-54
.
View Article PubMed Google Scholar -
Wu
C.,
Chen
X.,
Cai
Y.,
Xia
J.,
Zhou
X.,
Xu
S.,
Risk Factors Associated With Acute Respiratory Distress Syndrome and Death in Patients With Coronavirus Disease 2019 Pneumonia in Wuhan, China. JAMA Internal Medicine.
2020;
180
(7)
:
934-43
.
View Article PubMed Google Scholar -
Zhang
P.,
Li
J.,
Liu
H.,
Han
N.,
Ju
J.,
Kou
Y.,
Long-term bone and lung consequences associated with hospital-acquired severe acute respiratory syndrome: a 15-year follow-up from a prospective cohort study. Bone Research.
2020;
8
(1)
:
8
.
View Article PubMed Google Scholar -
George
P.M.,
Wells
A.U.,
Jenkins
R.G.,
Pulmonary fibrosis and COVID-19: the potential role for antifibrotic therapy. The Lancet. Respiratory Medicine.
2020;
8
(8)
:
807-15
.
View Article PubMed Google Scholar -
Tang
N.,
Bai
H.,
Chen
X.,
Gong
J.,
Li
D.,
Sun
Z.,
Anticoagulant treatment is associated with decreased mortality in severe coronavirus disease 2019 patients with coagulopathy. Journal of Thrombosis and Haemostasis.
2020;
18
(5)
:
1094-9
.
View Article PubMed Google Scholar -
Li
D.-Y.,
Li
R.-F.,
Sun
D.-X.,
Pu
D.-D.,
Zhang
Y.-H.,
Mesenchymal stem cell therapy in pulmonary fibrosis: a meta-analysis of preclinical studies. Stem Cell Res Ther.
2021;
12
(1)
:
461
.
View Article Google Scholar -
Tzouvelekis
A.,
Paspaliaris
V.,
Koliakos
G.,
Ntolios
P.,
Bouros
E.,
Oikonomou
A.,
A prospective, non-randomized, no placebo-controlled, phase Ib clinical trial to study the safety of the adipose derived stromal cells-stromal vascular fraction in idiopathic pulmonary fibrosis. J Transl Med.
2013;
11
:
171
.
View Article PubMed Google Scholar -
Averyanov
A.,
Koroleva
I.,
Konoplyannikov
M.,
Revkova
V.,
Lesnyak
V.,
Kalsin
V.,
First-in-human high-cumulative-dose stem cell therapy in idiopathic pulmonary fibrosis with rapid lung function decline. Stem Cells Translational Medicine.
2020;
9
(1)
:
6-16
.
View Article PubMed Google Scholar -
Campo
A.,
González-Ruiz
J.M.,
Andreu
E.,
Alcaide
A.B.,
Ocón
M.M.,
De-Torres
J.,
Endobronchial autologous bone marrow-mesenchymal stromal cells in idiopathic pulmonary fibrosis: a phase I trial. ERJ Open Res.
2021;
7
(2)
:
00773-2020
.
View Article PubMed Google Scholar -
Vu
N.B.,
Van Pham
P.,
Umbilical cord-derived mesenchymal stem cell transplantation for COVID-19 patients: long-term benefits for lung regeneration. Biomedical Research and Therapy.
2022;
9
(2)
:
4950-2
.
View Article Google Scholar -
Vu
N.B.,
Van Pham
P.,
Umbilical Cord Tissue-derived Mesenchymal Stem Cells Should be Considered as Adjuvant Therapy for COVID-19 Treatment: An Opinion from Pooled Clinical Evidence. Biomedical Research and Therapy.
2021;
8
(9)
:
4583-95
.
View Article Google Scholar -
Pham
P.V.,
Vu
N.B.,
Off-the-shelf mesenchymal stem cells from human umbilical cord tissue can significantly improve symptoms in COVID-19 patients: an analysis of evidential relations. World Journal of Stem Cells.
2020;
12
(8)
:
721-30
.
View Article PubMed Google Scholar -
Dong
L.-H.,
Jiang
Y.-Y.,
Liu
Y.-J.,
Cui
S.,
Xia
C.-C.,
Qu
C.,
The anti-fibrotic effects of mesenchymal stem cells on irradiated lungs via stimulating endogenous secretion of HGF and PGE2. Sci Rep.
2015;
5
:
8713
.
View Article PubMed Google Scholar -
Crestani
B.,
Marchand-Adam
S.,
Quesnel
C.,
Plantier
L.,
Borensztajn
K.,
Marchal
J.,
Hepatocyte growth factor and lung fibrosis. Proceedings of the American Thoracic Society.
2012;
9
(3)
:
158-63
.
View Article PubMed Google Scholar -
Cahill
E.F.,
Kennelly
H.,
Carty
F.,
Mahon
B.P.,
English
K.,
Hepatocyte growth factor is required for mesenchymal stromal cell protection against bleomycin-induced pulmonary fibrosis. Stem Cells Translational Medicine.
2016;
5
(10)
:
1307-18
.
View Article PubMed Google Scholar -
Chu
K.A.,
Yeh
C.C.,
Kuo
F.H.,
Lin
W.R.,
Hsu
C.W.,
Chen
T.H.,
Comparison of reversal of rat pulmonary fibrosis of nintedanib, pirfenidone, and human umbilical mesenchymal stem cells from Wharton's jelly. Stem Cell Research & Therapy.
2020;
11
(1)
:
513
.
View Article PubMed Google Scholar -
Cabrera
S.,
Gaxiola
M.,
Arreola
J.L.,
Ramírez
R.,
Jara
P.,
D'Armiento
J.,
Overexpression of MMP9 in macrophages attenuates pulmonary fibrosis induced by bleomycin. The International Journal of Biochemistry & Cell Biology.
2007;
39
(12)
:
2324-38
.
View Article PubMed Google Scholar -
Liang
J.,
Zhang
Y.,
Xie
T.,
Liu
N.,
Chen
H.,
Geng
Y.,
Hyaluronan and TLR4 promote surfactant-protein-C-positive alveolar progenitor cell renewal and prevent severe pulmonary fibrosis in mice. Nature Medicine.
2016;
22
(11)
:
1285-93
.
View Article PubMed Google Scholar -
Hofer
H.R.,
Tuan
R.S.,
Secreted trophic factors of mesenchymal stem cells support neurovascular and musculoskeletal therapies. Stem Cell Res Ther.
2016;
7
:
131
.
View Article PubMed Google Scholar -
J. Hou,
X. Peng,
J. Wang,
H. Zhang,
J. Xia,
Q. Ge,
X. Wang,
X. Chen,
X. Wu,
Mesenchymal stem cells promote endothelial progenitor cell proliferation by secreting insulin‑like growth factor‑1. Molecular Medicine Reports.
2017;
16
(2)
:
1502-1508
.
View Article PubMed Google Scholar -
Khan
P.,
Gazdhar
A.,
Savic
S.,
Lardinois
D.,
Roth
M.,
Tamm
M.,
118 Lung-derived mesenchymal stem cells exert anti-fibrotic effects in vitro. Chest.
2017;
151
(5)
:
15
.
View Article Google Scholar -
Xiao
K.,
He
W.,
Guan
W.,
Hou
F.,
Yan
P.,
Xu
J.,
Mesenchymal stem cells reverse EMT process through blocking the activation of NF-κB and Hedgehog pathways in LPS-induced acute lung injury. Cell Death & Disease.
2020;
11
(10)
:
863
.
View Article PubMed Google Scholar -
Zhang
E.,
Geng
X.,
Shan
S.,
Li
P.,
Li
S.,
Li
W.,
Exosomes derived from bone marrow mesenchymal stem cells reverse epithelial-mesenchymal transition potentially via attenuating Wnt/β-catenin signaling to alleviate silica-induced pulmonary fibrosis. Toxicology Mechanisms and Methods.
2021;
31
(9)
:
655-66
.
View Article PubMed Google Scholar -
Rahulan
V.,
Shah
U.,
Kumar
S.,
Ravipati
S.,
Dutta
P.,
Attawar
S.,
Mesenchymal Stem Cell Therapy for Patients with Post COVID ARDS on ECMO Referred for Lung Transplantation - Single Center Experience from India. The Journal of Heart and Lung Transplantation.
2022;
41
(S4)
:
S429-S430
.
View Article Google Scholar -
Vu
N.B.,
Phi
L.T.,
Dao
T.T.,
Le
H.T.,
Ta
V.T.,
Pham
P.V.,
Adipose derived stem cell transplantation is better than bone marrow mesenchymal stem cell transplantation in treating hindlimb ischemia in mice. Biomedical Research and Therapy.
2016;
3
(9)
:
1-13
.
View Article Google Scholar -
Liu
A.M.,
Chen
B.L.,
Yu
L.T.,
Liu
T.,
Shi
L.L.,
Yu
P.P.,
Human adipose tissue- and umbilical cord-derived stem cells: which is a better alternative to treat spinal cord injury?. Neural Regeneration Research.
2020;
15
(12)
:
2306-17
.
View Article PubMed Google Scholar -
Van Pham
P.,
Bich
N.V.,
Phan
N.K.,
Umbilical cord-derived stem cells (MODULATISTTM) show strong immunomodulation capacity compared to adipose tissue-derived or bone marrow-derived mesenchymal stem cells. Biomedical Research and Therapy.
2016;
3
(6)
:
1-10
.
View Article Google Scholar
Comments
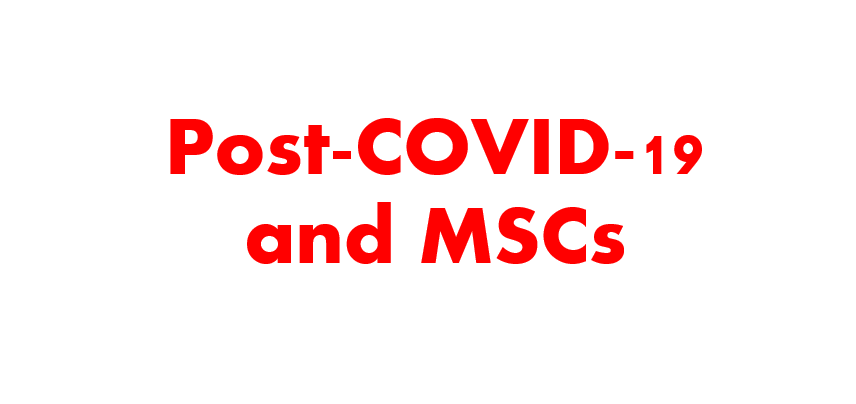
Article Details
Volume & Issue : Vol 9 No 5 (2022)
Page No.: 5075-5083
Published on: 2022-05-31
Citations
Copyrights & License

This work is licensed under a Creative Commons Attribution 4.0 International License.
Search Panel
Pubmed
Google Scholar
Pubmed
Google Scholar
Pubmed
Search for this article in:
Google Scholar
Researchgate
- HTML viewed - 5012 times
- PDF downloaded - 1328 times
- XML downloaded - 0 times