Abstract
Introduction: Many clinical studies have demonstrated that continuous exposure to pesticides, especially organophosphates and pyrethroids, causes toxicities such as carcinogenicity and neurotoxicity that lead to disorders such as diabetes, lung cancer, and neurodegenerative diseases. The mechanism underlying pesticide-induced neurotoxicity involves the production of ROS, which causes neuronal injury through oxidative stress.
Methods: In the present study, the neuronal SHSY5Y cell line was used to investigate the effect of the pesticides chlorpyrifos (organophosphate), aldicarb (carbamate), and deltamethrin (pyrethroid) on ROS-mediated toxicity and the protective effect of alpha-2-macroglobulin (a2M), a protease inhibitor and beta-amyloid plaque scavenger in the human brain. For cell viability and cytotoxicity, the MTT assay was performed. To monitor ROS production, assays such as DCFHDA, H2O2, and MDA were performed, along with assays of the activity of antioxidant enzymes such as superoxide dismutase and glutathione peroxidase.
Results: The experimental findings suggest a cytoprotective role for a2M in ROS-mediated toxicity that causes neuronal injury in humans.
Conclusion: Hence, a2M could be possibly used as a protective agent against oxidative neurotoxicity caused by pesticides.
Introduction
The pesticides chlorpyrifos (CPF), aldicarb (ALD), and deltamethrin (DLM; Figure 1) are known to cause both acute and chronic neurotoxicity in humans and animals through the inhibition of cholinesterase (ChE) activity in the synaptic junction of neurons, binding to gamma-aminobutyric acid (GABA)-gated channels by preventing the closure of sodium channels1. Humans are exposed to these pesticides in a number of ways, such as the consumption of fruits and vegetables contaminated with pesticide sprays and the inhalation of fumigants2, 3, 4, 5. Apart from causing substantial toxicity, these pesticides interfere with normal neuronal processes in vivo, leading to oxidative damage and cellular inflammation in the human brain6, 7, 8. Some of the modifications caused by these pesticides include oxidative stress9, disrupted neurotransmission10 and neuronal differentiation11, inhibition of replication in neuronal cells12, cognitive changes, decrease in psychomotor functions13, and apoptosis in neuronal cells by their metabolites14, 15, 16, 17. Such changes in neurons may lead to various neurodegenerative diseases such as Alzheimer’s disease (AD) and/or Parkinson’s disease (PD)18, 19, 20.
Alpha-2-macroglobulin (α2M) is produced in the human brain and keeps a check on reactive oxygen species (ROS)21 and ß-amyloid plaque formation, thereby preventing neuronal degeneration and AD progression. α2M is a serum protease inhibitor that may be involved in AD in mediating the clearance and degradation of Aß, a major constituent of ß-amyloid deposits22, 23. α2M, an acute-phase protein and a major component of the innate immune system, is a cerebrospinal fluid (CSF) marker of neuronal injury in preclinical AD22. An increased concentration of α2M in the blood is associated with neuronal injury24. Previous studies have shown that α2M interacts with the above mentioned pesticides3, 21, indicating the probable role of α2M as an unexplored biomarker in pesticide-induced ROS-mediated neurotoxicity25. Hence, in the present study, α2M was used to study the modulating/impairing effect of pesticide generated ROS on the neuronal SH-SY5Y (NCCS, Pune) cell line26.
Methods
Materials
All pesticides (CPF, ALD, and DLM) and chemicals (MTT dye, DMSO, pyrogallol, DMEM and Ham’s F12 medium, fetal bovine serum [FBS], thiobarbituric acid [TBA], 1% penicillin-streptomycin, dichlorodihydrofluorescein diacetate [DCFH-DA], glutathione [GSH], and NADPH) were purchased from Merck-Sigma (St. Louis, MO, USA). All other reagents used were of analytical standard.
Methods
Purification and characterization of human α2M
The α2M protein was isolated and purified from human blood plasma using ammonium sulfate precipitation followed by gel exclusion chromatography as per the method described previously3. A 5% (w/v) native PAGE was performed21 and the gel was stained with Coomassie brilliant blue R-250 (0.15% in 10% acetic acid). The gel was de-stained for 12 h in the de-staining solution (10% acetic acid), and the purified α2M formed a single band on the gel.
Cell culture
The SH-SY5Y cell line was cultured in a medium containing 1:1 DMEM and Ham’s F12 medium, 10% FBS, and 1% penicillin-streptomycin26. The cells were treated with a standard solution of pesticides and α2M, accordingly, to perform the experiments. Cells were used at 3–7 passages. The cells were divided into five groups based on the treatment with pesticides and proteins to obtain results for various stress markers. Group I was the control group comprising only SH-SY5Y cells under standard conditions (37 °C and 5% CO2). Group II consisted of SH-SY5Y cells incubated with α2M. The concentration of protein was 2 μM and the incubation time was 3 h (37 °C). Group III comprised pesticide (CPF, ALD, and DLM)-treated SH-SY5Y cells. Cells were treated with 5 μM of each pesticide (CPF, ALD, and DLM) separately for 3 h under standard conditions (37 °C and 5% CO2). Group IV was the pesticides (CPF, ALD, and DLM) and α2M group, in which the SH-SY5Y cells were treated with 5 μM of the pesticides for 3 h and then treated with α2M for 3 h.
Cell viability and proliferation assay
To determine the cytotoxicity and cell viability of the SH-SY5Y cells, the 3-(4, 5-dimethylthiazol-2-yl)-2, 5-diphenyltetrazolium bromide MTT assay was performed in 96-well plates27. The cells were used at 70 – 80% confluence. Later, the medium was removed and the cells were treated with pesticides (CPF, ALD, and DLM; 5 μM) for 6 h and later incubated with α2M for 3 h. Afterward, the cells were treated with MTT solution (5 mg/mL stock solution) at a final concentration of 0.5 mg/mL MTT. The cells were incubated for 4 h at 37 °C in 5% CO2. Finally, the cells were treated with 100 μL DMSO for 10 min to dissolve the formazan crystals. The absorbance was measured using an ELISA plate reader at 570 nm with a reference wavelength of 630 nm and was directly proportional to the number of viable cells. The experiments were performed in triplicate.
Measurement of ROS
ROS production was measured using DCFH-DA28. The cells were treated with α2M and the pesticides, following which 10 μM DCFH-DA was added to the medium for 1 h at 37 °C for diffusion into the cells. A multi-detection microplate reader was used for fluorescence measurement. The excitation and emission wavelengths for DCFH-DA were 485 and 535 nm, respectively28, 29, 30.
Measurement of malonaldehyde (MDA) levels
To detect the generation of ROS by pesticides in the neuronal cell line, the MDA levels were measured. The MDA levels in SH-SY5Y cells were quantified with the TBA reaction. Thiobarbituric acid reactive substances (TBARS) were measured by comparing the absorption to the standard curve of MDA equivalents generated by the acid-catalyzed hydrolysis of tetramethoxypropane31. The absorbance was recorded at 532 nm.
Measurement of superoxide dismutase (SOD) activity
The antioxidant enzyme SOD converts superoxide (O2-.) into H2O2 and O2, which is converted to water by other enzymes. The measurement was carried out as described previously32. To 80 μL of the cell suspension, 2.82 ml of 0.05 mM tris-succinate buffer was added, and the sample was treated for 30 min in a CO2 incubator. The reaction was initiated by adding 100 μL of 8 mM pyrogallol solution to each well32. The absorbance was read at 420 nm.
Measurement of glutathione peroxidase (GPx) levels
GPx are cytosolic enzymes that catalyze the conversion of H2O2 into H2O and O2 and the reduction of peroxide radicals (ROO.) into alcohol and oxygen. GPx levels were measured by determining the decrease in absorbance at 340 nm upon the oxidation of NADPH to NADP+ 33.
Statistically analysis
All experiments were repeated thrice and the data shown is the mean +- SD. P-value < 0.05 is considered as significant difference.
Enzyme | Control | CPF | ALD | DLM |
---|---|---|---|---|
SOD | 1.6 ± 0.140 | 0.90 ± 0.086 | 1.4 ± 0.131 | 0.88 ± 0.041 |
GPx | 24.4 ± 2.25 | 18.3 ± 1.31 | 20 ± 0.100 | 16.3 ± 0.07 |
Results
Cell viability and cell cytotoxicity assay
The MTT assay was performed to assess cell viability and cell proliferation activity, in which the quantity of formazan is directly proportional to the number of viable cells. The SH-SY5Y cells treated with all three pesticides (CPF, ALD, and DLM) in group III showed reduced cell viability (p < 0.001; Figure 1). Almost no effect on viability was seen for cells treated with α2M (group II), while the effect of the pesticides appeared to be reversed in cells treated with pesticides after incubation with α2M (group IV).
Measurement of ROS levels
Figure 3 shows the ROS levels induced by the pesticides as monitored by DCFHDA in the control group of SH-SY5Y cells and all three pesticides in group III (p < 0.001). The increased fluorescence in group III as compared to the control group and α2M group indicates the production of ROS. On the contrary, group IV showed a reduction in the fluorescence intensity as compared to group III, indicating the defensive function of α2M against ROS production.
Measurement of MDA
Figure 4 shows the TBARS activity in the SH-SY5Y cells in various groups. The highest MDA production was recorded in group III, in which the cells were treated with pesticides. However, group II showed no TBARS production. Group IV (pesticides + cells + α2M), however, showed a reduction in the TBARS level as compared to group III. The MDA levels were normal in the control group and slightly reduced in the α2M group (p < 0.001). However, MDA levels were significantly decreased in the α2M + pesticides + cells group (p < 0.001) compared to the pesticides group. Hence, the protective effects of α2M were observed with respect to MDA levels due to pesticide-triggered ROS in SH-SY5Y cells.
Measurement of SOD levels and GPx activity
Figure 5 shows the effect of various treatments on SOD levels in the control and other groups. Group III (pesticides group) showed reduced SOD activity as compared to group I (control) and group II (α2M; p < 0.001), demonstrating the toxicity triggered by the pesticides. On the other hand, group IV (α2M + pesticides + SH-SY5Y cells) showed a remarkable increase in SOD and GPx activity levels, indicating the protective effect of α2M against the pesticides.Table 1 shows the effect of pesticides on the activity of antioxidant enzymes in the cells. The pesticides group showed reduced GPx activity as compared to the control group I and α2M group II, while group IV (α2M and pesticides) showed increased GPx activity levels.
Discussion
CPF, ALD, and DLM are potent neurotoxic pesticides that affect various neuronal processes involved in the synaptic transmission and growth of neurons34, 35, 36, 37. α2M is an acute-phase protein produced in the brain to keep ROS levels under control3, 21 and prevent amyloid plaque formation by dissolving them through receptor-mediated endocytosis. It also protects the brain from undergoing neuronal degeneration25. α2M is also a major component of the innate immune system and acts as a hallmark of neuronal injury21. The human brain synthesizes and secretes α2M upon stimulation with interleukin-6, which indicates that α2M is an acute-phase protein in the human CNS. In this study, we characterized ROS formed by CPF, ALD, and DLM in the SH-SY5Y cell line. Furthermore, we investigated the ability of α2M to modulate the neurotoxic effect of these pesticides. The cellular toxicity of these pesticides on SH-SY5Y cells was first measured by the MTT assay, which indicated the toxic effects of the pesticides on the cell line.
DCFH-DA is a fluorimetric dye that is applied to quantify ROS38. This non-fluorescent compound diffuses into the cells, where it is sequentially hydrolyzed to release DCFH as the substrate by intracellular enzymes. ROS oxidize DCFH and convert it into DCF, a fluorescence product that is measured by spectrofluorometry using a microplate reader. A significant increase in the production of DCF was seen in pesticide-treated cells. This increased production of ROS results in oxidative damage to cellular components. However, when α2M was incubated with pesticide-treated cells, a contrary effect was observed. Similarly, regarding MDA levels, pesticide-treated groups showed a significant increase in absorbance, but in group IV, a decrease in absorbance was observed, suggesting the potential effect of α2M on pesticide-treated cells, in contradiction with results obtained for group IV. Regarding antioxidant enzymes, SOD and GPx activities were quantified at 420 nm and 340 nm, respectively, and reported as units per milligram of cells for SOD and nanomoles per minute per milligram of cells for GPx39. The results obtained for groups III, IV, and V suggest a cytoprotective role for α2M against ROS-mediated toxicity induced by the pesticides. Because proteases and ROS work through a common mechanism of inflammation, like in diseases such as atherosclerosis40, these findings also indicate the protective role of α2M in preventing damage to various cellular species by ROS apart from trapping proteases.
Conclusions
Our data showed that the selected pesticides were potent neurotoxic agents, while α2M decreased the cytotoxic effect of these pesticides. Hence, this study showed that α2M modulates pesticide-induced ROS-based neurotoxicity based on different parameters. Thus, α2M could possibly be used as a protective agent against neurotoxicity caused by ROS and pesticides, warranting further experimental studies.
Abbreviations
a2M: alpha-2-macroglobulin, ALD: aldicarb, CPF: chlorpyrifos, DCFHDA: dichlorodihydrofluorescein diacetate, DLM: deltamethrin, GPx: glutathione peroxidase, H2O2: hydrogen peroxide, MDA: malondialdehyde, melonaldehyde, ROS: reactive oxyhen species, SOD: superoxide dismutase, TBA: thiobarbituric acid, TBARS: thiobarbituric acid reactive substances
Acknowledgments
Research facilities provided by Department of Biochemistry, Aligarh Muslim University are gratefully acknowledged.
Author’s contributions
SD: data collection, analysis and interpretation of results; drafted the manuscript.
Funding
SD is thankful to Indian Council of Medical Research (ICMR), New Delhi for an ICMR-SRF fellowship to support her research experiments.
Availability of data and materials
Not applicable.
Ethics approval and consent to participate
Not applicable.
Consent for publication
Not applicable.
Competing interests
The authors declare that they have no competing interests.
References
-
Abou-Donia
M.B.,
Lapadula
D.M.,
Mechanisms of organophosphorus ester-induced delayed neurotoxicity: type I and type II. Annual Review of Pharmacology and Toxicology.
1990;
30
(1)
:
405-40
.
View Article PubMed Google Scholar -
Imam
A.,
Sulaiman
N.A.,
Oyewole
A.L.,
Chengetanai
S.,
Williams
V.,
Ajibola
M.I.,
Chlorpyrifos- and Dichlorvos-Induced Oxidative and Neurogenic Damage Elicits Neuro-Cognitive Deficits and Increases Anxiety-Like Behavior in Wild-Type Rats. Toxics.
2018;
6
(4)
:
71
.
View Article PubMed Google Scholar -
Dixit
S.,
Zia
M.K.,
Siddiqui
T.,
Ahsan
H.,
Khan
F.H.,
Interaction of organophosphate pesticide chlorpyrifos with alpha-2-macroglobulin: biophysical and molecular docking approach. Journal of Immunoassay & Immunochemistry.
2021;
42
(2)
:
138-53
.
View Article PubMed Google Scholar -
Jokanović
M.,
Neurotoxic effects of organophosphorus pesticides and possible association with neurodegenerative diseases in man: A review. Toxicology.
2018;
410
:
125-31
.
View Article PubMed Google Scholar -
Michaelis
S.,
Burdon
J.,
Vyvyan Howard
C.,
Aerotoxic Syndrome: A New Occupational Disease?. Public Health Panorama.
2017;
3
:
198-211
.
-
Lee
J.E.,
Park
J.H.,
Shin
I.C.,
Koh
H.C.,
Reactive oxygen species regulated mitochondria-mediated apoptosis in PC12 cells exposed to chlorpyrifos. Toxicology and Applied Pharmacology.
2012;
263
(2)
:
148-62
.
View Article PubMed Google Scholar -
Gupta
S.C.,
Mishra
M.,
Sharma
A.,
Deepak Balaji
T.G.,
Kumar
R.,
Mishra
R.K.,
Chlorpyrifos induces apoptosis and DNA damage in Drosophila through generation of reactive oxygen species. Ecotoxicology and Environmental Safety.
2010;
73
(6)
:
1415-23
.
View Article PubMed Google Scholar -
Chen
R.,
Cui
Y.,
Zhang
X.,
Zhang
Y.,
Chen
M.,
Zhou
T.,
Chlorpyrifos Induction of Testicular-Cell Apoptosis through Generation of Reactive Oxygen Species and Phosphorylation of AMPK. Journal of Agricultural and Food Chemistry.
2018;
66
(47)
:
12455-70
.
View Article PubMed Google Scholar -
Ki
Y.W.,
Park
J.H.,
Lee
J.E.,
Shin
I.C.,
Koh
H.C.,
JNK and p38 MAPK regulate oxidative stress and the inflammatory response in chlorpyrifos-induced apoptosis. Toxicology Letters.
2013;
218
(3)
:
235-45
.
View Article PubMed Google Scholar -
Slotkin
T.A.,
Seidler
F.J.,
Prenatal chlorpyrifos exposure elicits presynaptic serotonergic and dopaminergic hyperactivity at adolescence: critical periods for regional and sex-selective effects. Reproductive Toxicology (Elmsford, N.Y.).
2007;
23
(3)
:
421-7
.
View Article PubMed Google Scholar -
Yang
D.,
Howard
A.,
Bruun
D.,
Ajua-Alemanj
M.,
Pickart
C.,
Lein
P.J.,
Chlorpyrifos and chlorpyrifos-oxon inhibit axonal growth by interfering with the morphogenic activity of acetylcholinesterase. Toxicology and Applied Pharmacology.
2008;
228
(1)
:
32-41
.
View Article PubMed Google Scholar -
Qiao
D.,
Seidler
F.J.,
Slotkin
T.A.,
Developmental neurotoxicity of chlorpyrifos modeled in vitro: comparative effects of metabolites and other cholinesterase inhibitors on DNA synthesis in PC12 and C6 cells. Environmental Health Perspectives.
2001;
109
(9)
:
909-13
.
View Article PubMed Google Scholar -
Eaton
D.L.,
Daroff
R.B.,
Autrup
H.,
Bridges
J.,
Buffler
P.,
Costa
L.G.,
Review of the toxicology of chlorpyrifos with an emphasis on human exposure and neurodevelopment. Critical Reviews in Toxicology.
2008;
38
(sup2)
:
1-125
.
View Article PubMed Google Scholar -
Yu
F.,
Wang
Z.,
Ju
B.,
Wang
Y.,
Wang
J.,
Bai
D.,
Apoptotic effect of organophosphorus insecticide chlorpyrifos on mouse retina in vivo via oxidative stress and protection of combination of vitamins C and E. Experimental and Toxicologic Pathology.
2008;
59
(6)
:
415-23
.
View Article PubMed Google Scholar -
Li
Q.,
Kobayashi
M.,
Kawada
T.,
Chlorpyrifos induces apoptosis in human T cells. Toxicology.
2009;
255
(1-2)
:
53-7
.
View Article PubMed Google Scholar -
Park
J.H.,
Lee
J.E.,
Shin
I.C.,
Koh
H.C.,
Autophagy regulates chlorpyrifos-induced apoptosis in SH-SY5Y cells. Toxicology and Applied Pharmacology.
2013;
268
(1)
:
55-67
.
View Article PubMed Google Scholar -
Caughlan
A.,
Newhouse
K.,
Namgung
U.,
Xia
Z.,
Chlorpyrifos induces apoptosis in rat cortical neurons that is regulated by a balance between p38 and ERK/JNK MAP kinases. Toxicological Sciences.
2004;
78
(1)
:
125-34
.
View Article PubMed Google Scholar -
Yan
D.,
Zhang
Y.,
Liu
L.,
Yan
H.,
Pesticide exposure and risk of Alzheimer’s disease: A systematic review and meta-analysis. Sci Rep.
2016;
6
:
32222
.
View Article PubMed Google Scholar -
Jiang
X.,
Ganesan
P.,
Rengarajan
T.,
Choi
D.K.,
Arulselvan
P.,
Cellular phenotypes as inflammatory mediators in Parkinson's disease: interventional targets and role of natural products. Biomedicine and Pharmacotherapy.
2018;
106
:
1052-62
.
View Article PubMed Google Scholar -
Sánchez-Santed
F.,
Colomina
M.T.,
Herrero Hernández
E.,
Organophosphate pesticide exposure and neurodegeneration. Cortex.
2016;
74
:
417-26
.
View Article PubMed Google Scholar -
Dixit
S.,
Zia
M.K.,
Siddiqui
T.,
Ahsan
H.,
Khan
F.H.,
Interaction of Human Alpha-2-Macroglobulin with Pesticide Aldicarb Using Spectroscopy and Molecular Docking. Protein and Peptide Letters.
2021;
28
(3)
:
315-22
.
View Article PubMed Google Scholar -
Blacker
D.,
Wilcox
M.A.,
Laird
N.M.,
Rodes
L.,
Horvath
S.M.,
Go
R.C.,
Alpha-2 macroglobulin is genetically associated with Alzheimer disease. Nature Genetics.
1998;
19
(4)
:
357-60
.
View Article PubMed Google Scholar -
Kovacs
D.M.,
α2-macroglobulin in late-onset Alzheimer's disease. Experimental Gerontology.
2000;
35
(4)
:
473-9
.
View Article PubMed Google Scholar -
Varma
V.R.,
Varma
S.,
An
Y.,
Hohman
T.J.,
Seddighi
S.,
Casanova
R.,
Alpha-2 macroglobulin in Alzheimer's disease: a marker of neuronal injury through the RCAN1 pathway. Molecular Psychiatry.
2017;
22
(1)
:
13-23
.
View Article PubMed Google Scholar -
Dixit
S.,
Ahsan
H.,
Khan
F.H.,
Pesticides and plasma proteins: unexplored dimensions in neurotoxicity. International Journal of Pest Management.
2021;
:
1-10
.
View Article Google Scholar -
Baek
S.H.,
Park
T.,
Kang
M.G.,
Park
D.,
Anti-Inflammatory Activity and ROS Regulation Effect of Sinapaldehyde in LPS-Stimulated RAW 264.7 Macrophages. Molecules (Basel, Switzerland).
2020;
25
(18)
:
4089
.
View Article PubMed Google Scholar -
Mosmann
T.,
Rapid colorimetric assay for cellular growth and survival: application to proliferation and cytotoxicity assays. Journal of Immunological Methods.
1983;
65
(1-2)
:
55-63
.
View Article PubMed Google Scholar -
LeBel
C.P.,
Ischiropoulos
H.,
Bondy
S.C.,
Evaluation of the probe 2',7'-dichlorofluorescin as an indicator of reactive oxygen species formation and oxidative stress. Chemical Research in Toxicology.
1992;
5
(2)
:
227-31
.
View Article PubMed Google Scholar -
Wang
H.,
Joseph
J.A.,
Quantifying cellular oxidative stress by dichlorofluorescein assay using microplate reader. Free Radical Biology {&}amp; Medicine.
1999;
27
(5-6)
:
612-6
.
View Article PubMed Google Scholar -
Gay
C.,
Gebicki
J.M.,
A critical evaluation of the effect of sorbitol on the ferric-xylenol orange hydroperoxide assay. Analytical Biochemistry.
2000;
284
(2)
:
217-20
.
View Article PubMed Google Scholar -
Hasanzadeh
D.,
Mahdavi
M.,
Dehghan
G.,
Charoudeh
H.N.,
Farnesiferol C induces cell cycle arrest and apoptosis mediated by oxidative stress in MCF-7 cell line. Toxicology Reports.
2017;
4
:
420-6
.
View Article PubMed Google Scholar -
Marklund
S.,
Marklund
G.,
Involvement of the superoxide anion radical in the autoxidation of pyrogallol and a convenient assay for superoxide dismutase. European Journal of Biochemistry.
1974;
47
(3)
:
469-74
.
View Article PubMed Google Scholar -
Flohé
L.,
Günzler
W.A.,
Assays of glutathione peroxidase. Methods in Enzymology.
1984;
105
:
114-21
.
View Article PubMed Google Scholar -
Aktar
M.W.,
Sengupta
D.,
Chowdhury
A.,
Impact of pesticides use in agriculture: their benefits and hazards. Interdisciplinary Toxicology.
2009;
2
(1)
:
1-12
.
View Article PubMed Google Scholar -
Andersson
H.,
Tago
D.,
Treich
N.,
A review of evidence on health effects valuation of risks and benefit-cost analysis. Toulouse School of economics. Pestic Health.
2014;
24
:
203-95
.
-
de Oliveira
M.R.,
Peres
A.,
Ferreira
G.C.,
Schuck
P.F.,
Bosco
S.M.,
Carnosic Acid Affords Mitochondrial Protection in Chlorpyrifos-Treated Sh-Sy5y Cells. Neurotoxicity Research.
2016;
30
(3)
:
367-79
.
View Article PubMed Google Scholar -
Green
D.R.,
Reed
J.C.,
Mitochondria and apoptosis. Science.
1998;
281
(5381)
:
1309-12
.
View Article PubMed Google Scholar -
Keller
A.,
Mohamed
A.,
Dröse
S.,
Brandt
U.,
Fleming
I.,
Brandes
R.P.,
Analysis of dichlorodihydrofluorescein and dihydrocalcein as probes for the detection of intracellular reactive oxygen species. Free Radical Research.
2004;
38
(12)
:
1257-67
.
View Article PubMed Google Scholar -
Wasi
S.,
Tabrez
S.,
Ahmad
M.,
Toxicological effects of major environmental pollutants: an overview. Environmental Monitoring and Assessment.
2013;
185
(3)
:
2585-93
.
View Article PubMed Google Scholar -
Slack
M.A.,
Gordon
S.M.,
Protease Activity in Vascular Disease. Arteriosclerosis, Thrombosis, and Vascular Biology.
2019;
39
(10)
:
e210-8
.
View Article PubMed Google Scholar
Comments
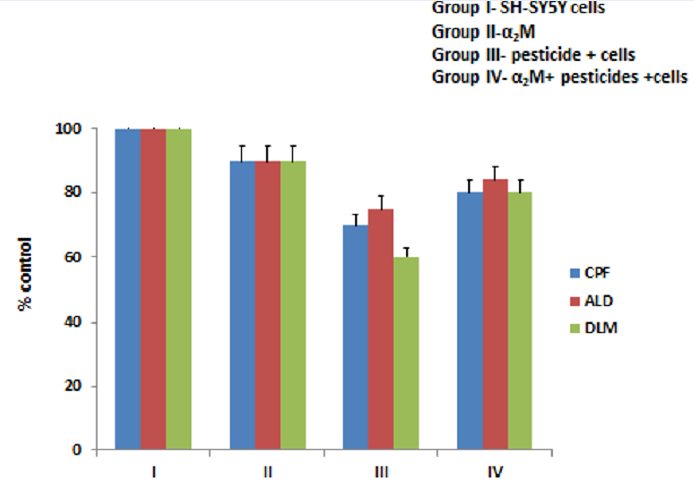
Article Details
Volume & Issue : Vol 10 No 2 (2023)
Page No.: 5537-5544
Published on: 2023-02-28
Citations
Copyrights & License

This work is licensed under a Creative Commons Attribution 4.0 International License.
Search Panel
Pubmed
Google Scholar
Pubmed
Google Scholar
Pubmed
Search for this article in:
Google Scholar
Researchgate
- HTML viewed - 3371 times
- PDF downloaded - 1054 times
- XML downloaded - 84 times