Abstract
Introduction: The presence of weaknesses in the efficacy of endogenous natriuretic and vasodilator agents plays a significant role in developing high blood pressure. It is often suggested that oxidative stress is critical in developing hypertension due to nitric oxide synthase (NOS) inhibition. This study aimed to investigate the intrarenal dopaminergic system activities, involvement of oxidative stress, and blood pressure changes resulting from NOS inhibition with N-nitro-L-arginine (L-NNA) and/or L-buthionine sulfoximine (BSO).
Methods: Male Wistar albino rats (n = 24) were administered water containing 50 mg/L L-NNA for 21 days and/or intraperitoneal injections of BSO (125 mg/kg twice daily) for seven days; control rats were administered tap water. The rats' blood pressure; water and salt balance; total oxidant and antioxidant capacities; and urinary dopamine, adrenaline, and noradrenaline levels were measured.
Results: While L-NNA and BSO alone did not significantly alter blood pressure, their coadministration caused rats to develop hypertension and significantly reduced the fractional excretion of sodium, increasing its tubular reabsorption. Urinary dopamine levels, indicators of intrarenal dopamine synthesis, did not change significantly.
Conclusion: These results indicate the importance of the weakness of endogenous natriuretic systems such as nitric oxide in hypertension development. While BSO did not induce oxidative stress in the measured parameters, it was shown for the first time as an actor in hypertension development in subjects with NOS inhibition to the extent that such inhibition did not increase blood pressure.
Introduction
The global prevalence of hypertension is increasing daily, contributing to the pathology of cardiovascular diseases and clinical complications. However, solution-oriented strategies for preventing and treating hypertension currently remain limited1. Nitric oxide (NO) is an important endogenous mediator influencing blood pressure regulation and local blood flow due to its potent vasodilator and anti-adhesive activity. It is synthesized from L-arginine by NO synthase (NOS) enzymes2, 3. Chronic inhibition of NOS isoforms by N-nitro-L-arginine (L-NNA) or its methyl ester N-ω-nitro-1-arginine methyl ester (L-NAME) leads to severe dose-related hypertension and cardiac dysfunction and remodeling4, 5, 6. The increase in blood pressure caused by acute and chronic NPS inhibition results from water and salt retention and increased vascular resistance7, 8. When the primary role of the kidneys in regulating long-term blood pressure levels was investigated, it appeared that renal sympathetic loading, oxidative stress, and NO deficiency induced hypertension9, 10, 11, 12.
Reactive oxygen species, which increase due to oxidative stress, may lead directly to hypertension development and unregulated redox reactions. In this context, oxidative stress can cause endothelial damage, vascular dysfunction, cardiovascular remodeling, renal dysfunction, sympathetic nervous system stimulation, and immune cell activation13, 14, 15. Oxidative stress may cause serious damage, especially to the endothelium, impairing endothelium-dependent vascular relaxation and increasing vascular contractile activity, leading to hypertension16. In hypertension, natriuresis, diuresis, and vasodilation, which are renal dopamine functions, are impaired10, 17. The presence of oxidative stress can impair the expression or function of the dopamine receptor in the kidney18, 19. The mechanism of this impairment of dopamine receptor function has yet to be fully elucidated. Oxidative stress, dopamine receptor dysfunction, and hypertension can coexist and are indeed interrelated20, 21. Despite the availability of studies on this subject, the mechanisms of NOS inhibition-induced hypertension and the involvement of oxidative stress have not yet been adequately clarified.
L-buthionine sulfoximine (BSO), an oxidant agent and gamma-glutamylcysteine synthetase enzyme inhibitor, has been found to cause severe hypertension in rats when administered at high doses and for a prolonged period22. Recent studies have shown that oxidative stress is also associated with high blood pressure and may eventually result in the progression of hypertensive complications23. However, it remains unknown whether L-NNA administered at a dose and duration that does not increase blood pressure alone significantly impacts blood pressure change, intrarenal dopamine synthesis ability, water-salt balance, and other renal functions when coadministered with BSO, which does cause oxidative stress alone. Therefore, blood pressure, water and salt balance, glomerular filtration rate (GFR), urine flow rate, sodium clearance (CNa), fractional excretion of sodium (FENa), tubular rejection fraction of sodium (TRFNa), total oxidant and antioxidant capacities, urinary dopamine, and adrenaline and noradrenaline levels were measured in subjects administered L-NNA with or without BSO at doses that would cause partial NOS inhibition. This study aimed to investigate the extent of oxidative stress and NO synthesis inhibition contributions to hypertension development.
Methods
This study was conducted at the Experimental Research Application and Research Centre (ÇOMÜDAM) of Çanakkale Onsekiz Mart University (ÇOMU) with the permission of the ÇOMU Local Ethics Committee of Animal Experiments.
Materials
This study included 24 male Wistar Kyoto rats with an average weight of 300 ± 15 g obtained from the ÇOMÜDAM Animal Laboratory. They were housed in standard rat cages under standard conditions (12 hours daylight/12 hours dark, ventilated, and constant temperature) with an equal number of rats per cage. They were given sufficient (ad libitum) water (tap water or water containing L-NNA) and feed (standard rat food containing 0.8% salt; Sigma-Aldrich, St. Louis, MO, USA) for 21 days. On the final day of the experiment, they were placed in individual metabolic cages.
L-NNA procedures
The L-NNA (98 TLC 2149-70-4; Sigma-Aldrich, St. Louis, MO, USA) was administered to the rats with drinking water. The rats were free to drink water in all groups. The L-NNA dose taken by the rats was calculated from the amount of water they drank. The L-NNA was prepared fresh at a concentration of 50 mg/L daily and administered to rats for 21 days8.
BSO procedure
BSO (97 TLC 83730-53-4; Sigma-Aldrich, St. Louis, MO, USA) was administered twice daily by intraperitoneal (IP) injection at a dose of 0.125 g/kg in a volume of 0.1 mL per 100 g weight for the final seven days. The rats were divided into four groups of six (n = 24). No medication was administered to rats in the control group. In the L-NNA group, the rats were administered L-NNA (50 mg/L) in drinking water for 21 days. In the control and L-NNA groups, rats were IP injected with distilled water (0.1 mL/100 g) for the final seven days. In the BSO group, rats were administered BSO (125mg/kg) in a volume of 0.1 mL/100 g via IP injection twice daily (morning and evening) for seven days. In the L-NNA+BSO group, the rats were administered L-NNA (50 mg/L) in drinking water for 21 days, and from the 14th day onwards, they were also administered BSO (125 mg/kg) in a volume of 0.1 mL/100 g via IP injection twice daily (morning and evening) for seven days.
Blood pressure measurements
The rats’ systolic blood pressure and heart rates were measured on the first and last day of the experiment (day 21) with the indirect tail-cuff method (MAY BPHR 9610-pc Tail-Cuff Indirect Blood Pressure Recorder; Ankara, Türkiye). Measurements were made in a quiet and calm laboratory environment after a rest and acclimatization period of approximately 20 minutes once the rat was relaxed and calm and a regular signal tone was received. The blood pressure measurements were recorded on the computer. The mean systolic blood pressure was calculated using three measurements from each rat.
Collection of urine samples
The rats were placed in metabolic cages on the final day of the experiment. The amounts of water they drank and urine they passed during 24 hours were recorded. Then, 0.1 mL of 6 N HCl was added to the containers for a 24-hour urine collection, with urine collected so that it was protected from sunlight. Urine samples were kept in Eppendorf tubes and stored at −80°C for biochemical measurements (Sanyo Ultra Low-Temperature Freezer Mdf-U4086S).
Collection of blood samples
At the end of the experiment, a sufficient amount of blood was taken directly from the rats’ hearts under light ether anesthesia.
Biochemical Analyses
Measurements of electrolytes (Na+, K+, Cl−), creatinine, and urea in serum and urine
The levels of electrolytes, urea, and creatinine were measured in serum and urine samples using the AutoAnalyzer (Roche Cobas 6000).
Measurement of urine dopamine, epinephrine, and norepinephrine levels
Epinephrine, norepinephrine, and dopamine were measured via a 24-hour urine collection procedure using an analytical column (Recipe, Germany) and an electrochemical detector (1049A) in the ultra-fast liquid chromatography (UFLC) system. Samples were prepared using a commercial kit according to its procedure (ClinRep, Munich, Germany). Briefly, 1.5 mL of acidified urine samples were placed into glass tubes, and 5 mL of reagent S was added. Next, 30 µL of the internal standard was added to each tube. Then, the pH was adjusted according to the kit’s procedure, and the samples were poured into the columns (Shim-pack HPLC; Shimadzu, Japan). After the liquid in the columns was completely filtered, the columns were washed with distilled water and placed in clean glass tubes. Then, 6 mL of reagent 2 was added to each column and allowed to be filtered. Dopamine, epinephrine, and norepinephrine levels in the obtained eluent were measured using the UFLC system.
Measurement of serum total oxidant/antioxidant capacity
Total oxidant status (TOS) and total antioxidant status (TAS) in serum were measured using the colorimetric method (Hitachi U1800 Spectrophotometer) and special kits (TOS and TAS Measurement Kits; RelAssay Diagnostics, Adana, Türkiye)24, 25.
Oxidative stress index (OSI)
The detected TOSs were multiplied by 100 and the TASs by 1000. The rats’ OSIs were calculated by dividing the adjusted TOS by the adjusted TAS.
Calculation of water balance
The rats’ water balance (water intake − urine excretion = water balance) was calculated by measuring their 24-hour water intake and urine output in the metabolic cages. Each rat’s CNa, GFR, FENa, and TRFNa were calculated using the formulas:
CNa = (urine sodium × urine flow rate) / plasma sodium
GFR = (urine creatinine / plasma creatinine) × urine flow rate
FENa = ([plasma creatinine × urine sodium] / [plasma sodium × urine creatinine]) × 100
TRFNa = ([urine sodium × urine volume] / [GFR × plasma sodium]) × 100
Daily sodium excretion and GFR, TRFNa, and FENa were calculated using these formulas.
Statistical analysis
Data obtained are presented as mean ± standard error. The data were compared between pairs of groups using Student’s t-test. Results with a P-value <0.05 were considered statistically significant.
First measured (mmHg) | Last measured (mmHg) | P | |
---|---|---|---|
CONTROL (n = 6) | 120.4 ± 4.82 | 126.7 ± 5.6 | 0.41 |
L-NNA (n = 6) | 132.5 ± 4.04 | 142.05 ± 5.7 | 0.20 |
133.3 ± 2.92 | 139.6 ± 6.1 | 0.38 | |
L-NNA+BSO (n = 5) | 121.5 ± 5.42 | 152.6 ± 8.1** | 0.01* |
Water intake (ml/day) | Urine output (ml/day) | Water balances (ml/day) | |
---|---|---|---|
CONTROL (n = 6) | 65.8 ± 2.2 | 36 ± 2.3 | 30.5 ± 1.8 |
L-NNA (n = 6) | 47.4 ± 3.8 **+ | 20.6 ± 2.6 ** | 26.3 ± 1.4 |
BSO (n = 5) | 62 ± 3.01 | 29.6 ± 4.5 | 34 ± 3.1 |
L-NNA+BSO (n = 5) | 44 ± 4.8 ** + | 24.4 ± 2.03 ** | 19.6 ± 3.8 **+ |
Serum sodium concentrations (mEq/l) | Urine sodium concentrations (mEq/l) | |
---|---|---|
Control (n = 6) | 147.3 ± 1.4 | 7.3 ± 1.3 |
L-NNA (n = 6) | 144 ± 0.9 | 3.2 ± 0.4 |
BSO (n = 5) | 146.6 ± 0.7 | 4.5 ± 1.1 |
L-NNA+BSO (n = 5) | 147 ± 0.44 # | 2.6 ± 0.4 **# |
CNa | GFR | FENa | TRFNa | |
---|---|---|---|---|
Control (n = 6) | 0.035 ± 0.006 | 2.8 ± 0.38 | 1.55 ± 0.4 | 1.44 ± 0.8 |
L-NNA (n = 6) | 0.015 ± 0.002 ** | 2.78 ± 0.29 | 0.5 ± 0.1 | 0.55 ± 0.1 |
BSO (n = 5) | 0.02 ± 0.005 | 3.25 ± 0.36 | 0.7 ± 0.3 | 0.66 ± 0.3 |
L-NNA+BSO (n = 5) | 0.012 ± 0.002 ** | 3.39 ± 0.41 | 0.3 ± 0.04 ** | 0.37 ± 0.1 ** |
Norepinephrine (µg/L) | Epinephrine (µg/L) | Dopamine (µg/L) | |
---|---|---|---|
CONTROL (n = 6) | 20.25 ± 3.96 | 230.5 ± 24.34 | 63.66 ± 10.44 |
L-NNA (n = 6) | 28.83 ± 5.09 | 446.66 ± 40.71 ** | 130.5 ± 30.39 |
BSO (n = 5) | 85 ± 36.76 | 214.6 ± 74.93 | 32.5 ± 9.97 # |
L-NNA+BSO (n = 5) | 127.4 ± 40.7 | 264.75 ± 69.1 | 299.5 ± 87.7 |
TOS μmol H 2 O 2 Eq/L | TAS μmol Trolox Eq/L | OSI | |
---|---|---|---|
Control (n = 6) | 9.38 ± 1.45 | 1.12 ± 0.11 | 0.89 ± 0.42 |
L-NNA (n = 6) | 17.94 ± 3.93 | 0.72 ± 0.10 | 2.84 ± 1.89 |
BSO (n = 5) | 9.13 ± 3.34 | 0.95 ± 0.06 | 0.98 ± 0.84 |
L-NNA+BSO (n = 5) | 5.9 ± 0.48 # | 1.02 ± 0.14 | 0.6 ± 0.12 # |
Results
Blood pressure
The rats’ baseline blood pressures did not differ significantly between the groups (Table 1). When L-NNA or BSO was administered alone, neither affected blood pressure compared to the initial blood pressure. However, when coadministered, they increased blood pressure significantly compared to the initial blood pressure (n = 5; 121.54 ± 5.42 vs. 152.64 ± 8.09; P < 0.05; Table 1) and the control group (n = 6; 126.7 ± 5.6; P < 0.05).
Effects on water intake, urine amount, and water balance
L-NNA and L-NNA+BSO administration significantly reduced water intake compared to the other groups. L-NNA+BSO coadministration decreased water intake compared to BSO administration alone and the control group (P < 0.05). No significant difference was detected in 24-hour water intake compared to the L-NNA group (Table 2). L-NNA and L-NNA+BSO administration significantly decreased the 24-hour urine volume compared to the control group (P < 0.05; Table 2). While the administration of either L-NNA or BSO alone did not change the water balance, their coadministration significantly decreased it (P < 0.05; Table 2).
Serum and urine sodium values
L-NNA+BSO administration significantly decreased the 24-hour urinary excretion of sodium compared to the control group (P < 0.05; Table 3).
CNa, GFR, and FENa
L-NNA and L-NNA+BSO administration significantly reduced the CNa, FENa, and TRFNa compared to the control group (P < 0.05; Table 4). The percentage of sodium that failed to be reabsorbed in the distal tubule was reduced. L-NNA+BSO coadministration increased sodium uptake (Table 4).
Urine dopamine, norepinephrine, and epinephrine concentrations
Urinary dopamine levels were significantly lower in the BSO group than in the L-NNA group (P < 0.05). While urinary dopamine, norepinephrine, and epinephrine levels increased in the L-NNA+BSO group, the increase was nonsignificant (Table 5). The urinary epinephrine concentration was significantly higher in the L-NNA group than in the control group (P < 0.05; Table 5).
OSI
OSIs were higher in the L-NNA administered group (n = 6; 2.84 ± 1.89) than in the L-NNA+BSO group but did not differ significantly from the control group (Table 6).
Discussion
This study investigated the effects of L-NNA, a NOS inhibitor, and BSO, a gamma-glutamyl-cysteine synthase inhibitor, on blood pressure, water and salt balance, sodium excretion, and urinary dopamine levels. The groups’ water balance graphs revealed that only L-NNA+BSO coadministration significantly decreased the water balance and increased blood pressure (Table 1, Table 2). The renal and endothelial systems are known to protect against the increase in blood pressure via diuresis and natriuresis in the organism18, 19, 26. However, in this study, natriuresis and diuresis did not occur despite the increased blood pressure due to combined L-NNA and BSO administration. Nevertheless, urinary 24-hour Na excretion, CNa, TRFNa, and FENa decreased significantly compared to the control group. Given such data, we can assume that increased sodium reabsorption (Na retention) causes increased blood pressure. Many relevant studies have reported that increased salt retention is responsible for the increased blood pressure resulting from prolonged low-dose NOS inhibition27, 28, 29. However, research has shown that intravenous (IV) L-NAME administration at 37.1 nmol/kg per minute for 11 days increased blood pressure without significantly changing sodium and water balance30. Another study showed that while there was no change in blood pressure when 50 ng/kg/min of L-NAME was administered by IV infusion for three days, the GFR and water and salt retention decreased27. L-NNA administration (50 mg/L) in drinking water for 21 days did not significantly change blood pressure, water-salt balance, CNa, TRFNa, FENa, and GFR. However, while coadministration of L-NNA at the same dose and duration with BSO (125 mg/kg) for the last seven days did not change the GFR, it was shown here for the first time that they significantly increased blood pressure but decreased CNa, TRFNa, and FENa, increasing tubular sodium reabsorption (Table 4).
NO plays a critical role in the long-term regulation of blood pressure and the control of renal functions. It has been suggested that partial and total blockade of the L-arginine-NO metabolic pathway increases renal and systemic vascular resistance and sympathetic nervous system activity but decreases renal blood flow, urine amount, and sodium excretion, increasing blood pressure7, 31, 32. The involvement of oxidative stress in hypertension development is often emphasized, although the exact mechanism has yet to be explored33, 34, 35, 36. Some reports state that superoxide anions (O−2), which cause oxidative stress, reduce renal blood flow and prevent sodium excretion31, 37. Furthermore, free oxygen radical scavengers (antioxidants) have been shown to reduce blood pressure significantly in different hypertension models, especially in salt-sensitive models32, 35, 38, 39. This study observed increased blood pressure after chronic NOS inhibition for three weeks when L-NNA was administered in drinking water. Oral treatment with lisinopril, one of the standard treatments, or sodium thiosulfate (STS), an experimental treatment, reduced lipid peroxidation in the urine, one indicator of systemic oxidative stress, by preventing the increase in blood pressure. In addition to their vasodilatory properties, such antioxidant effects of lisinopril and STS likely contribute to the cardioprotection observed in this study4.
There is increasing evidence that dopamine is an important regulator of kidney function and, ultimately, blood pressure10, 40. Dopamine is synthesized from levodopa, which is taken back from the tubules with sodium via the peripheral dopa decarboxylase enzymes. Dopa decarboxylase activity increases with high sodium diet intake but decreases with low sodium diet intake. There is particular evidence that changes in dietary salt and intracellular sodium modulate intrarenal dopamine synthesis/release. It is now well-known that intrarenal dopamine is one of the essential endogenous natriuretic systems10, 19. The intrarenal activity of dopamine depends on the activation of both D1- and D2-like dopamine receptors40, 41. Sodium reabsorption into the body occurs in the kidneys via the Na+/K+ ATPase and Na+/H+ exchange pumps. Dopamine inhibits the activities of these pumps in the proximal tubule. It has been shown that the dopamine D1 receptors and protein kinase C enzyme mediate this effect in the proximal tubule42.
Natriuresis, diuresis, and vasodilation, which are renal dopamine functions, are impaired in hypertension. Oxidative stress may impair the expression or function of the dopamine receptor in the kidney10, 20. The rats were administered BSO at 30 mmol/L to induce hypertension via an oxidative mechanism. However, its administration did not cause a significant increase in blood pressure or affect the GFR, but it decreased dopamine excretion while increasing oxidized dopamine excretion. Administering BSO with a high-salt diet has been reported to lead to increased blood pressure18. When these two different manipulative agents are coadministered, synergism and hypertension develop.
The oxidative stress efficiency of the BSO administered in this study was evaluated by calculating the TAS and TOS. However, TAS, TOS, and OSI values did not differ significantly between the BSO and control groups. These results did not appear to show the expected change in the groups, and a similar discordance was reported in another study8. For example, the TOS was 17.94 ± 3.93, and the OSI was >1 (n = 6; 2.84 ± 1.89) in the group administered only L-NNA. However, the OSI was <1 in the group coadministered BSO and the same L-NNA dose. Such coadministration of the oxidant agent BSO with L-NNA improved the antioxidant system but did not increase the TOS (n = 5; 5.9 ± 0.48) and even decreased the TOS significantly (Table 6). The method in question has certain inadequate and even inconsistent aspects in revealing oxidative stress.
While the increase in blood pressure was nonsignificant in the groups administered either L-NNA or BSO alone, the increased blood pressure when the two agents were coadministered indicated that partial NOS and gamma-glutamylcysteine synthase inhibition have a synergistic effect on blood pressure. Therefore, it can be stated that BSO accelerates the expected blood pressure increase with L-NNA administration. This study also observed that D1 receptor functions were impaired, and hypertension developed due to a high salt diet (1%) and administration of an oxidant agent (BSO) in rats. Furthermore, D1 receptor functions improved, and blood pressure returned to normal when the rats were administered SOD mimetic tempol43.
The ability to synthesize intrarenal dopamine can be evaluated by measuring the dopamine level in a 24-hour urine collection. This study measured the groups’ urine epinephrine, norepinephrine, and dopamine levels. While urinary dopamine levels did not differ significantly between the groups, they were higher in the L-NNA + BSO coadministered group than in the other groups. However, in this group, the coadministration of L-NNA and BSO significantly decreased CNa, TRFNa, and FENa, increasing tubular sodium reabsorption. It can be suggested that the increased tubular sodium reabsorption may be the reason for the emergence of increased blood pressure. In the general sense, it can also be assumed that oxidative stress is not only a cause but may also be a mediator of various mechanisms in hypertension development. High blood pressure is reportedly associated with decreased NO availability and increased oxidative stress and contributes to endothelial dysfunction, disruption of vascular relaxation, and disruption of the intrarenal dopaminergic system and natriuretic functions40, 44, 45.
One of this study’s most critical limitations is that it was a single-sex study. We aim to examine rats of both sexes in future studies. In addition, while oxidative stress was expected to develop with BSO and L-NNA coadministration, oxidative stress development was not observed with the parameters measured for that purpose. Moreover, intrarenal oxidative stress development and the deterioration of dopamine’s natriuretic function were also not revealed, which are additional limitations of this study. Further studies will be conducted that pay particular attention to these issues.
Conclusions
In this study, L-NNA and BSO coadministration did not affect the GFR but did decrease CNa, FENa, and TRFNa, increasing tubular sodium reabsorption, decreasing 24-hour urinary sodium excretion, and inducing hypertension. The study’s results showed that the weakness of endogenous natriuretic systems, such as NO and intrarenal dopamine, caused hypertension development. While intrarenal dopamine synthesis increased, it appeared that dopamine could not perform its natriuretic activity due to oxidative stress development. It was concluded that LNNA and BSO coadministration at a time and dose that did not increase blood pressure caused hypertension development.
Abbreviations
BSO: Buthionine sulfoximine; Can: Sodium clearance; FENa: fractional excretion of sodium; GFR: glomerular filtration rate; IP: intraperitoneal; L-NAME: N-ω-Nitro-1- Arginine methyl ester; L-NNA: Nw nitro-L-arginine; NO: Nitric oxide; NOS: nitric oxide synthase; OSI: oxidative stress index; TAS: total antioxidant Status; TOS: Total Oxidant Status; TRFNa: Tubular rejection fraction of sodium
Acknowledgments
The authors thank the Çanakkale Onsekiz Mart Univercity.
Author’s contributions
HEA, CS designed the research plan. The experimental process was carried out by ASA, BG. UHLPC, ELISA tests performed by ASA, BG, CS, HEA. The results of the study were evaluated and written by all researchers. All authors read and approved the final manuscript.
Funding
This study was supported by the Office of Scientific Research Projects at Çanakkale Onsekiz Mart University (project number: 2010/83).
Availability of data and materials
Data and materials used and/or analyzed during the current study are available from the corresponding author on reasonable request.
Ethics approval
The experiment was approved by the Local Ethics Committee of Animal Experiments at Çanakkale Onsekiz Mart University (Decision number 2009/9-2).
Consent for publication
Not applicable.
Competing interests
The authors declare that they have no competing interests.
References
-
Yu
J.,
Wang
S.,
Shi
W.,
Zhou
W.,
Niu
Y.,
Huang
S.,
Roxadustat prevents Ang II hypertension by targeting angiotensin receptors and eNOS. JCI Insight.
2021;
6
(18)
:
e133690
.
View Article PubMed Google Scholar -
Alderton
W.K.,
Cooper
C.E.,
Knowles
R.G.,
Nitric oxide synthases: structure, function and inhibition. The Biochemical Journal.
2001;
357
(Pt 3)
:
593-615
.
View Article PubMed Google Scholar -
Moncada
S.,
Nitric oxide: discovery and impact on clinical medicine. Journal of the Royal Society of Medicine.
1999;
92
(4)
:
164-9
.
View Article PubMed Google Scholar -
Nguyen
I.T.,
Wiggenhauser
L.M.,
Bulthuis
M.,
Hillebrands
J.L.,
Feelisch
M.,
Verhaar
M.C.,
Cardiac Protection by Oral Sodium Thiosulfate in a Rat Model of L-NNA-Induced Heart Disease. Frontiers in Pharmacology.
2021;
12
(April)
:
650968
.
View Article PubMed Google Scholar -
Biwer
L.A.,
Broderick
T.L.,
Xu
H.,
Carroll
C.,
Hale
T.M.,
Protection against L-NAME-induced reduction in cardiac output persists even after cessation of angiotensin-converting enzyme inhibitor treatment. Acta Physiologica (Oxford, England).
2013;
207
(1)
:
156-65
.
View Article PubMed Google Scholar -
Jin
S.,
Teng
X.,
Xiao
L.,
Xue
H.,
Guo
Q.,
Duan
X.,
Hydrogen sulfide ameliorated L-NAME-induced hypertensive heart disease by the Akt/eNOS/NO pathway. Experimental Biology and Medicine (Maywood, N.J.).
2017;
242
(18)
:
1831-41
.
View Article PubMed Google Scholar -
Vapaatalo
H.,
Mervaala
E.,
Nurminen
M.L.,
Role of endothelium and nitric oxide in experimental hypertension. Physiological Research.
2000;
49
(1)
:
1-10
.
PubMed Google Scholar -
Güngör
B.,
Akdur
A.S.,
S\ilan
C.,
Aksulu
H.E.,
\cSahin
O.,
The Effects of High Salt Diet and Exercise on the Water-Salt Balance and Blood Pressure in Rats. J Sci Perspect..
2021;
5
(1)
:
47-54
.
-
Harrison
D.G.,
Gongora
M.C.,
Oxidative stress and hypertension. The Medical Clinics of North America.
2009;
93
(3)
:
621-35
.
View Article PubMed Google Scholar -
Zeng
C.,
Villar
V.A.,
Yu
P.,
Zhou
L.,
Jose
P.A.,
Reactive oxygen species and dopamine receptor function in essential hypertension. Clinical and Experimental Hypertension (New York, N.Y.).
2009;
31
(2)
:
156-78
.
View Article PubMed Google Scholar -
Hurr
C.,
Young
C.N.,
Neural Control of Non-vasomotor Organs in Hypertension. Current Hypertension Reports.
2016;
18
(4)
:
30
.
View Article PubMed Google Scholar -
Tain
Y.L.,
Hsu
C.N.,
Oxidative Stress-Induced Hypertension of Developmental Origins: Preventive Aspects of Antioxidant Therapy. Antioxidants.
2022;
11
(3)
:
1-20
.
View Article PubMed Google Scholar -
Case
A.J.,
Tian
J.,
Zimmerman
M.C.,
Increased mitochondrial superoxide in the brain, but not periphery, sensitizes mice to angiotensin II-mediated hypertension. Redox Biology.
2017;
11
:
82-90
.
View Article Google Scholar -
Lopes
R.A.,
Neves
K.B.,
Tostes
R.C.,
Montezano
A.C.,
Touyz
R.M.,
Downregulation of Nuclear Factor Erythroid 2-Related Factor and Associated Antioxidant Genes Contributes to Redox-Sensitive Vascular Dysfunction in Hypertension. Hypertension.
2015;
66
(6)
:
1240-50
.
View Article PubMed Google Scholar -
Guzik
T.J.,
Touyz
R.M.,
Oxidative stress, inflammation, and vascular aging in hypertension. Hypertension.
2017;
70
(4)
:
660-7
.
View Article PubMed Google Scholar -
Grossman
E.,
Does increased oxidative stress cause hypertension? . Diabetes care.
2008;
31
(S2)
:
S185-9
.
View Article Google Scholar -
Ibarra
M.E.,
Borghese
M.F. Albertoni,
Majowicz
M.P.,
Ortiz
M.C.,
Loidl
F.,
Rey-Funes
M.,
Concerted regulation of renal plasma flow and glomerular filtration rate by renal dopamine and NOS I in rats on high salt intake. Physiological Reports.
2017;
5
(6)
:
1-12
.
View Article PubMed Google Scholar -
Banday
A.A.,
Lokhandwala
M.F.,
Renal Dopamine Oxidation and Inflammation in High Salt Fed Rats. Journal of the American Heart Association.
2020;
9
(1)
:
e014977
.
View Article PubMed Google Scholar -
Olivares-Hernández
A.,
Figuero-Pérez
L.,
Cruz-Hernandez
J.J.,
Sarmiento
R. González,
Usategui-Martin
R.,
Miramontes-González
J.P.,
Dopamine receptors and the kidney: an overview of health-and pharmacological-targeted implications. Biomolecules.
2021;
11
(2)
:
1-16
.
View Article PubMed Google Scholar -
Araujo
M.,
Wilcox
C.S.,
Oxidative stress in hypertension: role of the kidney. Antioxidants & Redox Signaling.
2014;
20
(1)
:
74-101
.
View Article PubMed Google Scholar -
Carlström
M.,
Wilcox
C.S.,
Arendshorst
W.J.,
Renal autoregulation in health and disease. Physiological Reviews.
2015;
95
(2)
:
405-511
.
View Article PubMed Google Scholar -
Vaziri
N.D.,
Wang
X.Q.,
Oveisi
F.,
Rad
B.,
Induction of oxidative stress by glutathione depletion causes severe hypertension in normal rats. Hypertension.
2000;
36
(1)
:
142-6
.
View Article PubMed Google Scholar -
Chen
J.R.,
Ko
J.,
Yeh
W.J.,
Huang
W.C.,
Yang
H.Y.,
Renoprotective effects of antroquinonol in rats with Nω-nitro-l-arginine methyl ester-induced hypertension. Nutrients.
2018;
10
(10)
:
1-10
.
View Article PubMed Google Scholar -
Erel
O.,
A new automated colorimetric method for measuring total oxidant status. Clinical Biochemistry.
2005;
38
(12)
:
1103-11
.
View Article PubMed Google Scholar -
Erel
O.,
A novel automated direct measurement method for total antioxidant capacity using a new generation, more stable ABTS radical cation. Clinical Biochemistry.
2004;
37
(4)
:
277-85
.
View Article PubMed Google Scholar -
Granger
J.P.,
Alexander
B.T.,
Abnormal pressure-natriuresis in hypertension: role of nitric oxide. Acta Physiologica Scandinavica.
2000;
168
(1)
:
161-8
.
View Article PubMed Google Scholar -
Salazar
F.J.,
Pinilla
J.M.,
López
F.,
Romero
J.C.,
Quesada
T.,
Renal effects of prolonged synthesis inhibition of endothelium-derived nitric oxide. Hypertension.
1992;
20
(1)
:
113-7
.
View Article PubMed Google Scholar -
Baylis
C.,
Harton
P.,
Engels
K.,
Endothelial derived relaxing factor controls renal hemodynamics in the normal rat kidney. Journal of the American Society of Nephrology.
;
1
(6)
:
875-81
.
View Article Google Scholar -
Yuasa
S.,
Li
X.,
Hitomi
H.,
Hashimoto
M.,
Fujioka
H.,
Kiyomoto
H.,
Sodium sensitivity and sympathetic nervous system in hypertension induced by long-term nitric oxide blockade in rats. Clinical and Experimental Pharmacology & Physiology.
2000;
27
(1-2)
:
18-24
.
View Article PubMed Google Scholar -
Manning
R.D.,
Hu
L.,
Mizelle
H.L.,
Montani
J.P.,
Norton
M.W.,
Cardiovascular responses to long-term blockade of nitric oxide synthesis. Hypertension.
1993;
22
(1)
:
40-8
.
View Article PubMed Google Scholar -
Usui
M.,
Egashira
K.,
Kitamoto
S.,
Koyanagi
M.,
Katoh
M.,
Kataoka
C.,
Pathogenic role of oxidative stress in vascular angiotensin-converting enzyme activation in long-term blockade of nitric oxide synthesis in rats. Hypertension.
1999;
34
(4 Pt 1)
:
546-51
.
View Article PubMed Google Scholar -
Kopkan
L.,
Majid
D.S.,
Superoxide contributes to development of salt sensitivity and hypertension induced by nitric oxide deficiency. Hypertension.
2005;
46
(4)
:
1026-31
.
View Article PubMed Google Scholar -
Welch
W.J.,
Blau
J.,
Xie
H.,
Chabrashvili
T.,
Wilcox
C.S.,
Angiotensin-induced defects in renal oxygenation: Role of oxidative stress. American Journal of Physiology-Heart and Circulatory Physiology.
2005;
288
(1)
:
H22-8
.
View Article Google Scholar -
Kopkan
L.,
Majid
D.S.A.,
Enhanced superoxide activity modulates renal function in NO-deficient hypertensive rats. Hypertension.
2006;
47
(3 II)
:
568-72
.
View Article Google Scholar -
Manning
R.D.,
Tian
N.,
Meng
S.,
Oxidative stress and antioxidant treatment in hypertension and the associated renal damage. American Journal of Nephrology.
2005;
25
(4)
:
311-7
.
View Article PubMed Google Scholar -
Silva
G.M. da,
Silva
M.C. da,
Nascimento
D.V.,
Silva
E.M. Lima,
Gouvêa
F.F.,
Lopes
L.G. de França,
Nitric oxide as a central molecule in hypertension: focus on the vasorelaxant activity of new nitric oxide donors. Biology (Basel).
2021;
10
(10)
:
1041
.
View Article PubMed Google Scholar -
Majid
D.S.A.,
Nishiyama
A.,
Jackson
K.E.,
Castillo
A.,
Inhibition of nitric oxide synthase enhances superoxide activity in canine kidney. American Journal of Physiology-Regulatory, Integrative and Comparative Physiology.
2004;
287
(1)
:
R27-32
.
View Article Google Scholar -
Beswick
R.A.,
Zhang
H.,
Marable
D.,
Catravas
J.D.,
Hill
W.D.,
Webb
R. Clinton,
Long-term antioxidant administration attenuates mineralocorticoid hypertension and renal inflammatory response. Hypertension.
2001;
37
(2 II)
:
781-6
.
View Article Google Scholar -
Hamza
S.M.,
Dyck
J.R.,
Systemic and renal oxidative stress in the pathogenesis of hypertension: modulation of long-term control of arterial blood pressure by resveratrol. Frontiers in Physiology.
2014;
5
(August)
:
292
.
View Article PubMed Google Scholar -
Yang
J.,
Villar
V.A.,
Jose
P.A.,
Zeng
C.,
Renal Dopamine Receptors and Oxidative Stress: role in Hypertension. Antioxidants & Redox Signaling.
2021;
34
(9)
:
716-35
.
View Article PubMed Google Scholar -
Carey
R.M.,
Theodore Cooper Lecture: Renal dopamine system: paracrine regulator of sodium homeostasis and blood pressure. Hypertension.
2001;
38
(3)
:
297-302
.
View Article PubMed Google Scholar -
Asghar
M.,
Hussain
T.,
Lokhandwala
M.F.,
Activation of dopamine D(1)-like receptor causes phosphorylation of α(1)-subunit of Na(+),K(+)-ATPase in rat renal proximal tubules. European Journal of Pharmacology.
2001;
411
(1-2)
:
61-6
.
View Article PubMed Google Scholar -
Banday
A.A.,
Lokhandwala
M.F.,
Inhibition of natriuretic factors increases blood pressure in rats. Am J Physiol -. American Journal of Physiology. Renal Physiology.
2009;
297
(2)
:
397-402
.
View Article Google Scholar -
Champlain
J. de,
Wu
R.,
Girouard
H.,
Karas
M.,
Midaoui
A. EL,
Laplante
M.A.,
Oxidative stress in hypertension. Clinical and Experimental Hypertension (New York, N.Y.).
2004;
26
(7-8)
:
593-601
.
View Article PubMed Google Scholar -
Ahmad
K.A.,
Yuan
D. Yuan,
Nawaz
W.,
Ze
H.,
Zhuo
C.X.,
Talal
B.,
Antioxidant therapy for management of oxidative stress induced hypertension. Free Radical Research.
2017;
51
(4)
:
428-38
.
View Article PubMed Google Scholar
Comments
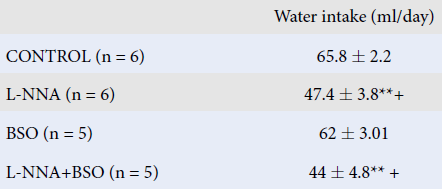
Article Details
Volume & Issue : Vol 10 No 6 (2023)
Page No.: 5735-5743
Published on: 2023-06-30
Citations
Copyrights & License

This work is licensed under a Creative Commons Attribution 4.0 International License.
Search Panel
Pubmed
Google Scholar
Pubmed
Google Scholar
Pubmed
Google Scholar
Pubmed
Google Scholar
Pubmed
Search for this article in:
Google Scholar
Researchgate
- HTML viewed - 2957 times
- PDF downloaded - 1095 times
- XML downloaded - 114 times