Abstract
Introduction: The interaction between the T cell immune checkpoint proteins, the programmed death-1 (PD-1) receptor, and its ligand PD-L1 plays a crucial role in T cell suppression and the evasion of cancer cells from immune detection, thereby promoting tumour growth. Nivolumab, a PD-1 inhibitor, disrupts this interaction, offering a potential therapeutic anti-cancer strategy. The goals of this study were to identify the optimal dosage of nivolumab that effectively decreases PD-1 protein expression in a mouse model, and to examine the impact on tumour growth.
Methods: We utilized a xenograft mouse model with EMT6 mammary carcinoma cells. Eight female Balb/C mice were inoculated with EMT6 cells and assigned to three groups: a control (n = 2) and two treatment groups receiving nivolumab at 5 mg/kg (n = 3) and 10 mg/kg (n = 3) on days 10, 12, and 14 postinoculation. Tumour sizes were measured at specified intervals using electronic callipers, and the mice were sacrificed on day 16 to assess PD-1 protein levels via sandwich ELISA.
Results: There was no significant difference in tumour volume across all groups compared to the controls. PD-1 protein expression was significantly lower in Group 3 (10 mg/kg nivolumab) than in both Group 2 and the control group.
Conclusion: Nivolumab administration at a dose of 10 mg/kg markedly reduced PD-1 protein expression in a tumour-bearing mouse model, suggesting that higher doses of nivolumab may be more effective in modulating immune responses against tumour growth. These findings contribute to our understanding of nivolumab's pharmacodynamics and underscore the importance of dose optimization in enhancing the therapeutic efficacy of the drug against cancer.
INTRODUCTION
Immune checkpoints are crucial in regulating the balance of activities involved in the immune response. The PD-1 receptor and its ligand, PD-L1, are immune T-cell checkpoints controlling the activation and maintenance of immune tolerance within the tumour microenvironment. The PD-1/PD-L1 signalling pathway prevents the activation of effector T lymphocytes, thus enhancing the immunological tolerance of tumour cells and ultimately leading to immune escape1.
As a consequence, tumour cells continue to proliferate and spread by evading detection and attack from the immune system2. The PD-1 protein is expressed by immune cells, including peripherally activated T- and B-cells, macrophages, and some dendritic cells3. The PD-1 receptors include PD-L1 and PD-L2. Both receptors are expressed in antigen-presenting cells (APC), including dendritic cells, macrophages and B cells4. PD-L1 also has been reported in tumour cells5. The activation of the PD-1 signalling pathway leads to decreased T cell proliferation and the production of cytokines such as IFN-γ, tumour necrosis factor-α, and interleukin-2 (IL-2), and thus affects cell survival6.
PD-1 regulates the activation of immune checkpoints by binding to its ligand PD-L1 and subsequently triggers an intracellular signalling cascade that halts the activation of the immune response, thereby regulating the release of cytokines by immune cells2. The binding of PD-1 with PD-L1 at the tumour surface in tumour infiltration lymphocytes has been proposed as the cause of the loss of lymphocyte function that consequently allows the tumour to escape the action of the immune system.
PD-1 and PD-L1 are also expressed at abnormally high levels by several forms of tumours such as lymphocytic leukaemia7, oral squamous carcinoma8, nasopharyngeal carcinoma9, breast cancer10, and melanoma and lung cancer2, indicating that these proteins are the primary components involved in enhancing the capacity of tumour immune escape8, 9, 10, 11. Thus, it is unclear whether PD-1 exerts a positive or negative effect in either eliminating the destructive immune responses or encouraging the proliferation of cancer cells through inhibition or exhaustion of tumour immunity5. Data on the antagonistic therapeutic impact of PD-1/PD-L1 on solid tumours are currently limited. Despite a 40 to 50% effectiveness of anti-PD-L1 antagonists, both anti-PD-1 and anti-PD-L1 medications have shown extremely poor efficacy against metastatic melanoma, lung, and colorectal cancers12. The poor treatment response may be linked to the complex involvement of the tumour microenvironment in the development and metastasis of cancer as well as the degree of genetic variation among patients2.
Radiation therapy stimulates a variety of immune cells to infiltrate the tumour microenvironment and promote antitumor responses13, 14, 15, 16 Nevertheless, immune escape has the opposite effect, allowing for tumour relapse17. Cytokines and tumour-derived exosomes in the tumour microenvironment can stimulate PD-L1 expression and promote tumour immune suppression2. Combining conventional radiation with anti-PD-1 demonstrated significant antitumor immunity in small-cell lung cancer18. The United States Food and Drug Administration (FDA) has currently approved the usage of several PD-1/PD-L1 inhibitors for cancer immunotherapy, including nivolumab, pembrolizumab, cemiplimab, atezolizumab, durvalumab, and avelumab, for the treatment of various types of solid tumours19, 20.
Our group has successfully developed an acquired gamma-ray radioresistant model, i.e., an EMT6 mouse-bearing mammary carcinoma using fractionated irradiation at 2 Gy / cycle. The development of radioresistant EMT6 cells occurred through the STAT/ AKT pathway via overexpression of the PD-1 protein. The current study investigated the effect of gamma-ray radiation combined with a PD-1 inhibitor (nivolumab) on the proliferation of radioresistant EMT6 cells in a mouse mammary carcinoma model. Nivolumab has been extensively studied for the treatment of glioblastoma21, adenocarcinoma22, and triple-negative breast cancer23. Nivolumab inhibited the binding of PDL-1 and PDL-2 to receptors in non-small-cell lung cancer (NSCLC) and renal cell carcinoma patients24.
MATERIALS AND METHODS
Cell Line
EMT6 (American Type Culture Collection, USA) is an epithelial cell line isolated from the breast of a mouse with a mammary tumour. The cells were cultured in Dulbecco’s Modified Eagle Medium (ThermoFisher Scientific, USA) supplemented with 10% fetal bovine serum, 100 µg/ml streptomycin, and 100 U/ml penicillin (CELLGRO Mediatech, USA) at 37°C in a humidified incubator with a 5% CO2 atmosphere. The cells were subcultured after reaching about 80% confluence. The cells used in the assays were maintained at least at the fourth passage.
Nivolumab
Nivolumab (MedChem Express, New Jersey) is a monoclonal antibody that inhibits the binding of PD-1 with its ligand PD-L1. A stock solution (10 mg/ml) was diluted accordingly with phosphate-buffered saline (PBS) to 5 mg/kg and 10 mg/kg before use.
Mouse tumour model
Ten-week-old female Balb/C mice were purchased from the Laboratory Animal Facility and Management (LAFAM), UiTM, and housed at the Laboratory Animal Care Unit, UiTM. The handling of mice was in accordance with protocols approved by LAFAM. The mouse tumour model was prepared according to Ibrahim et al. (2016)25. The left hind leg of a Balb/C mouse was shaved before inoculation with 1 x 106 EMT cells. The primary tumour was collected 15 days post-inoculation and processed for further investigation. All procedures were performed in accordance with Research Animal Ethic Committee UiTM (UITM CARE: 316/2020) guidelines.
Group | Reading 1 | Reading 2 | Reading 3 | Average concentration | Mean ± s.d |
Control | 195.74 | 186.3 | 203.72 | 195.25 | |
Group 1 (5 mg/kg) | 185.8 | 190.8 | 263.55 | 213.38 | 213.38±43.52 |
Group 2 (10 mg/kg) | 81.8 | 70.55 | 82.05 | 78.13 | 78.13±6.57 |
Nivolumab treatment and protein collection
Eight healthy, ten-week-old female Balb/C mice weighing approximately 18 to 22 grams were divided into three groups. Group 1 served as a control, while groups 2 and 3 were intravenously treated with nivolumab at 10 mg/kg and 5 mg/kg, respectively. The first dose of nivolumab was administered through the tail after the tumours were palpable or visible, which was approximately 10 days post-inoculation, and was followed by two additional doses on days 12 and 14. Nivolumab administration was based on a previous study26 with modification. The width and length of tumours were measured on days 1, 4, 8, 10, 14 and 16 post-inoculation using an electronic calliper. Tumours were harvested on day 16 post-inoculation and immediately weighed. End-point tumours were placed immediately in radioimmunoprecipitation assay (RIPA) buffer (ThermoFisher Scientific, USA) supplemented with protease inhibitor and stored at -80°C. The flowchart of the study design is summarized in Figure 1.
PD-1 protein extraction and measurement
The PD-1 protein was quantitatively measured using an Enzyme Linked-Immunosorbent Assay (ELISA) kit according to the manufacturer’s instructions (Bioassay Technology Laboratory, UK). The samples were washed with ice-cold PBS three or four times and agitated for two hours at 4°C. The supernatant was collected after centrifugation at 13,000 × g for 20 minutes. A 50 µL aliquot of each standard and sample was added into designated 96-microtiter wells (ThermoFisher Scientific, USA). The same volume of working solution was added and incubated for 45 minutes at 37°C. Then, 100 µL of HRP conjugate working solution was added and incubated for 30 minutes at 37°C. After that, 90 μL of the substrate reagent was added before a final incubation of 15 minutes at 37°C in the dark. The absorbance was read at 450 nm using a microplate reader after the addition of 50 μL of stop solution.
Statistical analysis
Statistical analysis was performed using GraphPad Prism 8.0. Data are presented as the mean and standard error. A non-parametric, unpaired t-test was performed for comparison between two groups, with the significance level set as P < 0.05.
RESULTS
Effect of different doses of Nivolumab on tumour growth
Tumour growth in mice inoculated with EMT6 cells was monitored by measuring the width and length of the tumours from day 1 to 16 post-inoculation. Beginning on day 10 after tumour inoculation, 0.2 mg (equivalent to 10 mg/kg) and 0.1 mg (equivalent to 5 mg/kg) of Nivolumab were injected intravenously through the tail vein using three doses, each given two days apart. The tumours developed gradually in both groups before the mice were sacrificed, and no significant difference was observed between the two doses of Nivolumab. Nonetheless, there was a trend showing that a 10 mg/kg dose of Nivolumab decreased tumour growth on Day 14 and Day 16 post-inoculation compared to a 5 mg/kg dose of Nivolumab. This suggested that 10 mg/kg may potentially affect tumour growth (Figure 2 ).
Protein concentration extracted from tumour section
Table 1 shows the protein concentration extracted from tumour sections in each group of mice. Group 2 which was injected with 10 mg/kg nivolumab showed the lowest protein concentration.
Effect of different doses of Nivolumab on PD-1 levels
The expression of the PD-1 protein in the tumours was characterized using the ELISA technique. Figure 3 shows the expression of PD-1 observed in tumours treated with 10 mg/kg and 5 mg/kg of nivolumab. The relative expression of PD-1 in mice given a 10 mg/kg dose of nivolumab decreased significantly compared to the control and 5 mg/kg dose groups (P < 0.05). This suggested that a 10 mg/kg dose of nivolumab could significantly reduce PD-1 expression.
DISCUSSION
In the present study, we analysed the effect of different concentrations of nivolumab on PD-1 protein expression on tumour growth. There are many mouse models that have been employed to study the development of cancer in the preclinical stage, including syngeneic mice, genetically modified mice, human xenograft mice, and patient xenograft humanized mice27. In this research, we used syngeneic Balb/C mice that were injected with EMT6 mouse mammary cancer, cells that have undergone numerous immunological alterations in the tumour microenvironment28, 29.
In this study, we introduced the nivolumab at three different time points on days 10, 12 and 14 post-inoculation, similar to other studies, although the type of cells, the type of anti-PD-1, and the dose chosen were different30, 31. Mathios et al. (2016)30 and Wu et al. (2019)31 exposed C57BL/6J mice to a PD-1 inhibitor on days 10, 12 and 14 after implantation of GL261 Luc cells. Additionally, other studies have introduced PD-1 inhibitors at three time points but on different days post-inoculation32, 33. A previous study introduced anti-PD-1 drugs at 5, 10 and 15 days after intracranial tumour inoculation of GL261 cells34. Another study treated mice with a PD-1 inhibitor on days 3, 6, and 9 after inoculation with GL261 cells. A study by Christine (2023) treated transgenic C57BL/6-h. PD-1 mice implanted with MC38 colon cancer using six doses of nivolumab given on days 0, 3, 6, 9, 12, and 15 post-inoculation35.
We observed that nivolumab treatment at a dose of 10 mg/kg resulted in less tumour growth than the control and 5 mg/kg groups, albeit this difference was not statistically significant. Only on day 14, two days after the first dosage of nivolumab, did the rate of tumour growth in Group 2 begin to decline. In the past, tumour ablation was observed in adenocarcinoma xenograft mice that received PD-1 monotherapy injections on days 7, 10, and 13. Nevertheless, this study tracked the tumour progression for 33 days22. This shows that nivolumab can slow tumour growth over a longer time of observation. This conclusion was also supported by the study of Reardon showing that the administration of a PD-1 blocker in an advanced intracranial glioblastoma tumour mouse model resulted in the mice remaining alive without evidence of tumour at more than 100 days after tumour implantation21.
To further investigate PD-1 activity, we measured the expression in the tumour microenvironment using ELISA after the mice were treated with nivolumab. In this case, Group 2 mice treated with 10 mg/kg nivolumab exhibited a significantly lower level of PD-1 than the 5 mg/kg nivolumab and control groups. Similarly, a study by Selby et al. found that when administering an anti-PD-1 monoclonal antibody (nivolumab) at 10 mg/kg, the addition of anti-CTLA-4 at a lower dose resulted in anti-tumour activity36. The latter study also observed that using anti-PD-1 as a single drug resulted in T-cell infiltration into tumours in MC38 and CT26 mouse colorectal tumour models36, suggesting that PD-1 was successfully blocked. Another study found that anti-PD-1 monotherapy, which prevented PD-1 interactions, caused small increases in CD8+ T cells in tumours that were responsive to the treatment37 and showed that IL-13 levels were also elevated with PD-1 treatment, supporting the hypothesis that IL-13 plays a role in anti-tumour activity37, 38.
CONCLUSION
This study showed that nivolumab at a dose of 10 mg/kg influenced tumour growth and PD-1 activity. However, this has only been found in a syngeneic tumour model. Further study is needed using non-responsive models.
Abbreviations
PD-1: Programmed Death-1, PD-L1: Programmed Death-Ligand 1, EMT6: Epithelial-Mesenchymal Transition 6, ELISA: Enzyme Linked-Immunosorbent Assay, APC: Antigen-Presenting Cells, IFN-γ: Interferon-gammaI, L-2: Interleukin-2, NSCLC: Non-Small Cell Lung Cancer, FDA: Food and Drug Administration, RIPA: Radioimmunoprecipitation Assay, PBS: Phosphate-Buffered Saline, LAFAM: Laboratory Animal Facility and Management, UiTM: Universiti Teknologi MARA, STAT: Signal Transducer and Activator of Transcription, AKT: Protein Kinase, BGL261 Luc cells: GL261 Luciferase cells, C57BL/6J mice: C57 Black 6 Jackson mice, MC38: Mouse Colon 38, CTLA-4: Cytotoxic T-Lymphocyte Antigen 4
Acknowledgments
The authors express their gratitude to the funders, Ministry of Higher Education Malaysia and Universiti Teknologi MARA for their support.
Author’s contributions
Funding acquisition: MJI, NAH, HHH; Conception: NRFS, MJI; Methodology: NFRS, NAH, MJI; Interpretation or analysis of data: NRFS, NAHH, MJI; Preparation of the manuscript: NH, NAHH, NH, MKAK, SBSAF, SO, NJO, MJI; Revision for important intellectual content: NFRS, HHH, NAHH, SO, NJO, SBSAF, EO, MJI; Supervision: NAHH, HHH, MKAK, MJI. All authors read and approved the final manuscript.
Funding
The study was partly funded by Fundamental Research Grant Scheme, Ministry of Education Malaysia (FRGS/1/2019/SKK08/UITM/02/9) and Lestari Research Grant, Universiti Teknologi MARA (600-RMC/MYRA 5/3/LESTARI (094/2020)).
Availability of data and materials
Data and materials used and/or analyzed during the current study are available from the corresponding author on reasonable request.
Ethics approval
All procedures were performed in accordance with Research Animal Ethic Committee UiTM (UITM CARE: 316/2020) guidelines.
Consent for publication
Not applicable.
Competing interests
The authors declare that they have no competing interests.
References
-
Chamoto
K.,
Al-Habsi
M.,
Honjo
T.,
Role of PD-1 in Immunity and Diseases. Current Topics in Microbiology and Immunology.
2017;
410
:
75-97
.
View Article PubMed Google Scholar -
Jiang
X.,
Wang
J.,
Deng
X.,
Xiong
F.,
Ge
J.,
Xiang
B.,
Role of the tumor microenvironment in PD-L1/PD-1-mediated tumor immune escape. Molecular Cancer.
2019;
18
(1)
:
10
.
View Article PubMed Google Scholar -
Zhai
Y.,
Moosavi
R.,
Chen
M.,
Immune Checkpoints, a Novel Class of Therapeutic Targets for Autoimmune Diseases. Frontiers in Immunology.
2021;
12
.
View Article PubMed Google Scholar -
Li
T.,
Ma
R.,
Zhu
J.Y.,
Wang
F.S.,
Huang
L.,
Leng
X.S.,
PD-1/PD-L1 costimulatory pathway-induced mouse islet transplantation immune tolerance. Transplantation Proceedings.
2015;
47
(1)
:
165-70
.
View Article PubMed Google Scholar -
Dermani
F.K.,
Samadi
P.,
Rahmani
G.,
Kohlan
A.K.,
Najafi
R.,
PD-1/PD-L1 immune checkpoint: potential target for cancer therapy. Journal of Cellular Physiology.
2019;
234
(2)
:
1313-25
.
View Article PubMed Google Scholar -
Riella
L.V.,
Paterson
A.M.,
Sharpe
A.H.,
Chandraker
A.,
Role of the PD-1 pathway in the immune response. American Journal of Transplantation.
2012;
12
(10)
:
2575-87
.
View Article PubMed Google Scholar -
Gamaleldin
M.A.,
Ghallab
O.M.,
Nadwan
E.A.,
Elwafa
R.A. Abo,
PD-1 and PD-L1 gene expressions and their association with Epstein-Barr virus infection in chronic lymphocytic leukemia. Clinical & Translational Oncology.
2021;
23
(11)
:
2309-22
.
View Article PubMed Google Scholar -
Lin
Y.M.,
Sung
W.W.,
Hsieh
M.J.,
Tsai
S.C.,
Lai
H.W.,
Yang
S.M.,
High PD-L1 Expression Correlates with Metastasis and Poor Prognosis in Oral Squamous Cell Carcinoma. PLoS One.
2015;
10
(11)
.
View Article PubMed Google Scholar -
Zhou
Y.,
Miao
J.,
Wu
H.,
Tang
H.,
Kuang
J.,
Zhou
X.,
PD-1 and PD-L1 expression in 132 recurrent nasopharyngeal carcinoma: the correlation with anemia and outcomes. Oncotarget.
2017;
8
(31)
:
51210-23
.
View Article PubMed Google Scholar -
Qin
T.,
Zeng
Y.D.,
Qin
G.,
Xu
F.,
Lu
J.B.,
Fang
W.F.,
High PD-L1 expression was associated with poor prognosis in 870 Chinese patients with breast cancer. Oncotarget.
2015;
6
(32)
:
33972-81
.
View Article PubMed Google Scholar -
Tang
Y.,
He
Y.,
Shi
L.,
Yang
L.,
Wang
J.,
Lian
Y.,
Co-expression of AFAP1-AS1 and PD-1 predicts poor prognosis in nasopharyngeal carcinoma. Oncotarget.
2017;
8
(24)
:
39001-11
.
View Article PubMed Google Scholar -
Sznol
M.,
Blockade of the B7-H1/PD-1 pathway as a basis for combination anticancer therapy. Cancer Journal (Sudbury, Mass.).
2014;
20
(4)
:
290-5
.
View Article PubMed Google Scholar -
Demaria
S.,
Golden
E.B.,
Formenti
S.C.,
Role of Local Radiation Therapy in Cancer Immunotherapy. JAMA Oncology.
2015;
1
(9)
:
1325-32
.
View Article PubMed Google Scholar -
Dovedi
S.J.,
Cheadle
E.J.,
Popple
A.L.,
Poon
E.,
Morrow
M.,
Stewart
R.,
Fractionated Radiation Therapy Stimulates Antitumor Immunity Mediated by Both Resident and Infiltrating Polyclonal T-cell Populations when Combined with PD-1 Blockade. Clinical Cancer Research.
2017;
23
(18)
:
5514-26
.
View Article PubMed Google Scholar -
Sharabi
A.B.,
Lim
M.,
DeWeese
T.L.,
Drake
C.G.,
Radiation and checkpoint blockade immunotherapy: radiosensitisation and potential mechanisms of synergy. The Lancet. Oncology.
2015;
16
(13)
:
e498-509
.
View Article PubMed Google Scholar -
Teresa Pinto
A.,
Laranjeiro Pinto
M.,
Patrícia Cardoso
A.,
Monteiro
C.,
Teixeira Pinto
M.,
Filipe Maia
A.,
Ionizing radiation modulates human macrophages towards a pro-inflammatory phenotype preserving their pro-invasive and pro-angiogenic capacities. Scientific Reports.
2016;
6
(1)
:
18765
.
View Article PubMed Google Scholar -
Barker
H.E.,
Paget
J.T.,
Khan
A.A.,
Harrington
K.J.,
The tumour microenvironment after radiotherapy: mechanisms of resistance and recurrence. Nature Reviews. Cancer.
2015;
15
(7)
:
409-25
.
View Article PubMed Google Scholar -
Gong
X.,
Li
X.,
Jiang
T.,
Xie
H.,
Zhu
Z.,
Zhou
F.,
Combined Radiotherapy and Anti-PD-L1 Antibody Synergistically Enhances Antitumor Effect in Non-Small Cell Lung Cancer. Journal of Thoracic Oncology.
2017;
12
(7)
:
1085-97
.
View Article PubMed Google Scholar -
Chen
S.,
Zhang
Z.,
Zheng
X.,
Tao
H.,
Zhang
S.,
Ma
J.,
Response Efficacy of PD-1 and PD-L1 Inhibitors in Clinical Trials: A Systematic Review and Meta-Analysis. Frontiers in Oncology.
2021;
11
.
View Article PubMed Google Scholar -
Tan
S.,
Zhang
C.W.,
Gao
G.F.,
Seeing is believing: anti-PD-1/PD-L1 monoclonal antibodies in action for checkpoint blockade tumor immunotherapy. Signal Transduction and Targeted Therapy.
2016;
1
(1)
:
16029
.
View Article PubMed Google Scholar -
Reardon
D.A.,
Gokhale
P.C.,
Klein
S.R.,
Ligon
K.L.,
Rodig
S.J.,
Ramkissoon
S.H.,
Glioblastoma Eradication Following Immune Checkpoint Blockade in an Orthotopic, Immunocompetent Model. Cancer Immunology Research.
2016;
4
(2)
:
124-35
.
View Article PubMed Google Scholar -
Juneja
V.R.,
McGuire
K.A.,
Manguso
R.T.,
LaFleur
M.W.,
Collins
N.,
Haining
W.N.,
PD-L1 on tumor cells is sufficient for immune evasion in immunogenic tumors and inhibits CD8 T cell cytotoxicity. The Journal of Experimental Medicine.
2017;
214
(4)
:
895-904
.
View Article PubMed Google Scholar -
Katano
I.,
Hanazawa
A.,
Otsuka
I.,
Yamaguchi
T.,
Mochizuki
M.,
Kawai
K.,
Development of a novel humanized mouse model for improved evaluation of in vivo anti-cancer effects of anti-PD-1 antibody. Scientific Reports.
2021;
11
(1)
:
21087
.
View Article PubMed Google Scholar -
Liu
J.,
Chen
Z.,
Li
Y.,
Zhao
W.,
Wu
J.,
Zhang
Z.,
PD-1/PD-L1 Checkpoint Inhibitors in Tumor Immunotherapy. Frontiers in Pharmacology.
2021;
12
.
View Article PubMed Google Scholar -
Ibahim
M.J.,
Yang
Y.,
Crosbie
J.C.,
Stevenson
A.,
Cann
L.,
Paiva
P.,
Eosinophil-associated gene pathways but not eosinophil numbers are differentially regulated between synchrotron microbeam radiation treatment and synchrotron broad-beam treatment by 48 hours postirradiation. Radiation Research.
2016;
185
(1)
:
60-8
.
View Article PubMed Google Scholar -
Selby
M.J.,
Engelhardt
J.J.,
Johnston
R.J.,
Lu
L.S.,
Han
M.,
Thudium
K.,
Preclinical development of ipilimumab and nivolumab combination immunotherapy: mouse tumor models, in vitro functional studies, and cynomolgus macaque toxicology. PLoS One.
2016;
11
(9)
.
View Article PubMed Google Scholar -
Sanmamed
M.F.,
Chester
C.,
Melero
I.,
Kohrt
H.,
Defining the optimal murine models to investigate immune checkpoint blockers and their combination with other immunotherapies. Annals of Oncology : Official Journal of the European Society for Medical Oncology.
2016;
27
(7)
:
1190-8
.
View Article PubMed Google Scholar -
Jin
Y.,
An
X.,
Mao
B.,
Sun
R.,
Kumari
R.,
Chen
X.,
Different syngeneic tumors show distinctive intrinsic tumor-immunity and mechanisms of actions (MOA) of anti-PD-1 treatment. Scientific Reports.
2022;
12
(1)
:
3278
.
View Article PubMed Google Scholar -
Yu
J.W.,
Bhattacharya
S.,
Yanamandra
N.,
Kilian
D.,
Shi
H.,
Yadavilli
S.,
Tumor-immune profiling of murine syngeneic tumor models as a framework to guide mechanistic studies and predict therapy response in distinct tumor microenvironments. PLoS One.
2018;
13
(11)
.
View Article PubMed Google Scholar -
Mathios
D.,
Kim
J.E.,
Mangraviti
A.,
Phallen
J.,
Park
C.K.,
Jackson
C.M.,
Anti–PD-1 antitumor immunity is enhanced by local and abrogated by systemic chemotherapy in GBM. Science translational medicine.
2016;
8
(370)
:
370ra180
.
View Article Google Scholar -
Wu
A.,
Maxwell
R.,
Xia
Y.,
Cardarelli
P.,
Oyasu
M.,
Belcaid
Z.,
Combination anti-CXCR4 and anti-PD-1 immunotherapy provides survival benefit in glioblastoma through immune cell modulation of tumor microenvironment. Journal of Neuro-Oncology.
2019;
143
(2)
:
241-9
.
View Article PubMed Google Scholar -
J. Dejaegher,
T. Verschuere,
E. Vercalsteren,
Boon
L.,
J. Cremer,
R. Sciot,
S.W. Van Gool,
S. De Vleeschouwer,
Characterization of PD‐1 upregulation on tumor‐infiltrating lymphocytes in human and murine gliomas and preclinical therapeutic blockade. International Journal of Cancer.
;
141
(9)
:
1891-900
.
View Article Google Scholar -
Dejaegher
J.,
Verschuere
T.,
Vercalsteren
E.,
Boon
L.,
Cremer
J.,
Sciot
R.,
Characterization of PD-1 upregulation on tumor-infiltrating lymphocytes in human and murine gliomas and preclinical therapeutic blockade. International Journal of Cancer.
2017;
141
(9)
:
1891-900
.
View Article PubMed Google Scholar -
Jahan
N.,
Talat
H.,
Alonso
A.,
Saha
D.,
Curry
W.T.,
Triple combination immunotherapy with GVAX, anti-PD-1 monoclonal antibody, and agonist anti-OX40 monoclonal antibody is highly effective against murine intracranial glioma. OncoImmunology.
2019;
8
(5)
.
View Article PubMed Google Scholar -
Bulaon
C.J.,
Sun
H.,
Malla
A.,
Phoolcharoen
W.,
Therapeutic efficacy of plant-produced Nivolumab in transgenic C57BL/6-hPD-1 mouse implanted with MC38 colon cancer. Biotechnology Reports (Amsterdam, Netherlands).
2023;
38
.
View Article PubMed Google Scholar -
Selby
M.J.,
Engelhardt
J.J.,
Johnston
R.J.,
Lu
L.S.,
Han
M.,
Thudium
K.,
Preclinical Development of Ipilimumab and Nivolumab Combination Immunotherapy: Mouse Tumor Models, In Vitro Functional Studies, and Cynomolgus Macaque Toxicology. PLoS One.
2016;
11
(9)
.
View Article PubMed Google Scholar -
Ngiow
S.F.,
Young
A.,
Jacquelot
N.,
Yamazaki
T.,
Enot
D.,
Zitvogel
L.,
A Threshold Level of Intratumor CD8+ T-cell PD1 Expression Dictates Therapeutic Response to Anti-PD1. Cancer Research.
2015;
75
(18)
:
3800-11
.
View Article PubMed Google Scholar -
Lebel-Binay
S.,
Laguerre
B.,
Quintin-Colonna
F.,
Conjeaud
H.,
Magazin
M.,
Miloux
B.,
Experimental gene therapy of cancer using tumor cells engineered to secrete interleukin-13. European Journal of Immunology.
1995;
25
(8)
:
2340-8
.
View Article PubMed Google Scholar
Comments
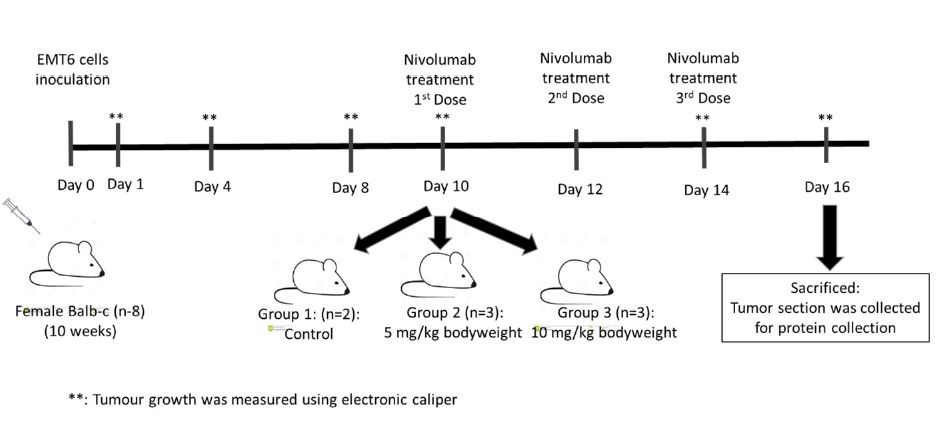
Article Details
Volume & Issue : Vol 11 No 2 (2024)
Page No.: 6191-6197
Published on: 2024-02-29
Citations
Copyrights & License

This work is licensed under a Creative Commons Attribution 4.0 International License.
Search Panel
- HTML viewed - 1257 times
- PDF downloaded - 446 times
- XML downloaded - 28 times