Abstract
Introduction: Glucagon-like peptide-1 (GLP-1) plays a critical role in glucose regulation by stimulating insulin secretion in a glucose-dependent manner. It enhances the functionality of pancreatic β-cells, minimizing apoptotic signals while promoting their proliferation and neogenesis. Consequently, leveraging plant-based GLP-1 agonists offers a promising approach to mitigating hyperglycemia in individuals with type 2 diabetes. This study aimed to explore the potential of methanol extract from Apium graveolens (commonly known as celery) to enhance insulin secretion, inhibit dipeptidyl peptidase-4 (DPP-4), and boost GLP-1 levels. Furthermore, it assessed celery's ability to protect pancreatic β cells against glucotoxicity and H2O2-induced toxicity in vitro. Additionally, we sought to identify the flavonoids present in the crude ethanolic extract of celery through HPLC/MS analysis.
Methods: We assessed cell viability, cell integrity (using lactate dehydrogenase (LDH) leakage assay), mitochondrial function (via ATP production), and insulin secretion in INS-1 pancreatic β-cells. GLP-1 activation and insulin sensitivity were evaluated in GLUTag cells, while DPP-4 activity was measured using an in vitro inhibitory assay.
Results: HPLC/MS analysis revealed the presence of 11 phenolic compounds in celery. Treatment with varying concentrations of celery extract (100, 125, 150, 200 µg/mL) resulted in decreased cell death, significantly improved cell viability, and increased cellular ATP levels, thereby offering protection against glucotoxicity and H2O2-induced toxicity. Additionally, it boosted insulin production and reduced insulin resistance. The inhibitory effect of celery extract on DPP-4 activity, coupled with the increase in GLP-1 halflife, enhances insulin secretion from β cells, outlining celery's potential hypoglycemic mechanism.
Conclusion: The findings of this study underscore celery's therapeutic potential as a treatment option for individuals with type 2 diabetes, attributing to its ability to modulate insulin secretion, enhance pancreatic β-cell survival, and inhibit DPP-4, thereby increasing GLP-1 levels. This illuminates a promising avenue for further research and potential diabetes management strategies.
Introduction
Type 2 diabetes mellitus is a metabolic disorder characterized by hyperglycemia and insulin resistance1. Inadequate insulin secretion from pancreatic β-cells and defective hepatic glucose production are involved in the progression of this disease. During the development of type 2 diabetes, pancreatic β-cell mass and function decrease, leading to research for new treatments to preserve β-cell function2. Significant evidence suggests that several herbal extracts might protect the function of pancreatic β-cells, increasing attention to the treatment of diabetes as alternative and complementary medicines.
Glucagon-like peptide-1 (GLP-1) is considered a gluco-incretin hormone that intervenes in insulin secretion, β-cell preservation and proliferation, maintains normal blood glucose levels, and enhances peripheral insulin levels3. However, GLP-1 is rapidly degraded by dipeptidyl peptidase-4 (DPP-4) in plasma within 1.5 minutes after its secretion, consequently having a very short half-life4. Recently, GLP-1 has been utilized for the management of type 2 diabetes, showing modest clinical effects, as it improves fasting glycemia and reduces postprandial glucose levels. Nonetheless, DPP-4 inhibitors play a significant role in prolonging the circulation half-life of GLP-1 by preventing its degradation, making them an attractive agent for the management of type 2 diabetes, which can ameliorate metabolic control without causing weight loss or severe hypoglycemia5.
Celery (Apium graveolens L.), belonging to the Apiaceae family, is frequently used in traditional medicine. Previous studies have demonstrated that celery possesses various pharmacological effects, such as improving the lipid profile in obese rats6, hepaprotective ability7, cytotoxic activity8, increasing the level of interleukin-10 and decreasing interleukin-1β, reducing neutrophil migration9, antiglycation, and antidiabetic effects10, as well as reducing cholesterol and triglycerides11. This study was carried out to assess the effects of methanol extracts from A. graveolens on INS-1 pancreatic β cells challenged by glucose and H2O2 and its effects on GLP-1, DPP-4, as well as their activity on insulin-resistant C2C12 skeletal muscle cells.
Methods
Chemicals and Reagents
3-(4,5-Dimethylthiazol-2-yl)-2,5-diphenyltetrazolium bromide (MTT) products were obtained from Sigma Chemical Co. (St. Louis, MO). GLUTag and INS-1 cells were acquired from Invitrogen (Carlsbad, CA, USA). RPMI-1640 (Gibco BRL, NY, USA), Dulbecco’s Modified Eagle Medium (DMEM), HEPES (pH 7.3), RPMI-1640 (Gibco BRL, NY, USA), GLP-1 Total ELISA kit, Millipore (Merck, USA), and Rat/mouse insulin was measured by ELISA (Millipore, Billerica, MA, U.S.A). RPMI medium, penicillin, and streptomycin were acquired from HyClone Laboratories (Logan, UT, USA).
Plant Material
Leaf samples were obtained from a cultivar in Amecameca, Mexico State, and a specimen was authenticated by botanist Aurora Chimal. A voucher specimen (6543) is stored in the Department of Botany of the Escuela Nacional de Ciencias Biologicas-IPN for preservation.
Plant Extraction
The samples were sliced into smaller pieces and then dried under shade at room temperature before being ground finely and subjected to extraction. One thousand grams of powder was extracted at reflux temperature for 4 h. The methanol extract was then filtered, and the process was repeated twice using the same quantity of solvent to extract the celery exhaustively. Methanol was removed from the extract using a rotavapor (BUCHI Rotavapor R-200, Switzerland) under reduced pressure at 45°C. The residues were combined and weighed (CEL) and calculated for percentage yield (19% w/w), then stored in a deep freeze. The methanol extract was reconstituted with distilled water for assays.
Characterization of Flavonoids
Flavonoids were characterized using a Shimadzu HPLC system (Kyoto, Japan), consisting of an LC-20AT pump, an SPD-M20A DAD detector, a CTO-10AS VP column oven, and a SIL-20A autoinjector. A 10 µL aliquot of each sample was eluted through a C18 column of Inertsil ODS-SP (4.6 mm×250 mm, 5 µm) at a column temperature of 28 °C and a flow rate of 0.8 mL/min. The mobile phase consisted of solvent A (0.1% formic acid) and solvent B (acetonitrile). The gradient elution program was as follows: 0–5 min, 5%–15% B; 5–25 min, 15%–30% B; 25–39 min, 30%–38% B; 39–47 min, 38%–55% B; 47–50 min, 55%–70% B; 50–56 min, 70%–75% B; 56–60 min, 75%–100% B; 60–65 min, 100%–5% B; 65–75 min, 5% B. Chromatograms were obtained at 350 nm, and UV diode array detection spectra were scanned from 190–800 nm. Identification of flavonoids from methanol extract was performed by HPLC-ESI-MS using a Thermo Ion trap mass spectrometer (Waltham, MA, USA) equipped with an electrospray ionization (ESI) interface. The ESI-MS was applied in positive modes, and the product ions from protonated molecular ions were further analyzed by MS/MS (MS2). Nitrogen was used as the drying and nebulizing gas. Other parameters consisted of a drying gas temperature of 350 °C, capillary voltage of 3.5 kV, capillary exit voltage of 120.4 V, cap exit offset voltage of 77.2 V, and a range of m/z 100–1000 for full-scan MS analysis.
Cell Culture
Cell lines INS-1 and GLUTag cells were cultured in DMEM consisting of 11.1 mmol/L D-glucose supplemented with 100 µg/mL streptomycin, 100 U/mL penicillin, 10% fetal bovine serum, 2 mmol/L L-glutamine, 10 mmol/L HEPES, 1 mmol/L 50 µmol/L mercaptoethanol, and sodium pyruvate, in 5% CO2 at 37˚C. C2C12 mouse muscle cells (myotubes) were obtained from the American Type Culture Collection (Manassas, VA, USA). To assay insulin-resistant cells, myotubes were preincubated with 5.5 mM glucose in DMEM for 2 h without FBS and supplemented with 1% BSA and then incubated for 18 h in Modified Eagle Medium (DMEM) without fetal bovine serum (FBS), with 5.5 mM glucose, 0.75 mM palmitate, 1% bovine serum albumin (BSA), and 200 mM insulin.
Cell Viability, Integrity, and Mitochondrial Function
INS-1 cells were incubated with 400, 700, 1000, 1250, 1500 µg/mL of CEL, and 250 µM H2O2 for 15 min, and cell viability was evaluated using the test 3-(4,5-dimethylthiazol-2-yl)-2,5-diphenyltetrazolium bromide (MTT; Sigma-Aldrich Co., St. Louis, MO, USA). A stimulation of 250 µM H2O2 reduced cell viability to ~68%. Thus, oxidative stress was induced by the addition of 200 µM H2O2 for 15 min. In another assay, INS-1 cells were incubated with 100, 125, 150, 200 µg/mL, and 250 µM H2O2 for 15 min. Cell integrity was measured by LDH assay, determined as lactate dehydrogenase released in the culture medium. Briefly, after treatment, 0.2 mL culture medium from each group was evaluated for LDH activity using a commercial kit (Cayman Chemical, MI, USA) according to the manufacturer’s instructions. Mitochondrial function was measured by cellular ATP analysis. The culture medium was supplemented with CEL (150, and 200 µg/mL) and maintained for 24 h before cellular ATP analysis. H2O2 (250 mM) was incorporated 2 or 4 h before the end of the experiment. Cellular ATP levels were then measured in both cells exposed to CEL and H2O2 using a fluorometric assay kit (Abcam, Boston, MA, USA) according to the manufacturer’s instructions.
Glucose-stimulated Insulin Secretion (GSIS)
Glucotoxicity was induced in INS-1 cells incubated for five days with glucose at a concentration of 5.5 mM and 30 mM. INS-1 cells (3 X 105 cells/well) were incubated with 30 mM glucose and 25, 50, or 100 µg/mL of CEL or GLP-1 (10 nM) for 48 h. After removing supernatants, they were replaced with a medium containing a mixture of FBS (2%) and glucose (3 mM) and incubated for 5 h. Immediately afterward, the INS-1 cells were stimulated with 5.5- or 30-mM glucose in Krebs–Ringer buffer (20 mM HEPES, pH 7.4, 5 mM NaHCO3, 1.2 mM KH2PO4, 1.2 mM MgSO4, CaCl2, KCl, 2.54 mM and 119 mM NaCl) for 65 min at 37 °C. The supernatant was used for the detection of insulin secretion, employing an Elisa Kit according to the manufacturer’s instructions (Lingo Research, Lingo, MO, USA). GLP-1 (10 nM) was used as a standard.
Insulin Secretion Stimulated by H2O2
INS-1 cells (3 X 105 cells/well) were seeded in 96-well microplates with 100 µL culture medium for a period of 24 h before the addition of CEL and/or H2O2. After 24 h of treatment with CEL (25, 50, and 100 µg/mL) and/or H2O2 (250 µM), the cell supernatant was removed and rinsed with phosphate-buffered saline. Insulin secretion (GSIS) was measured using an insulin enzyme-linked immunosorbent assay kit (Lingo Research, Lingo, MO, USA). Untreated cells served as a control.
Insulin-Resistant Cell Model
Myotubes were preincubated in 500 µL of DMEM with 5.5 mM glucose for 4 h and various concentrations (125, 150, 200 µg/mL) of CEL. Then, after 4 h of incubation, 500 µL of the supernatant was collected, and the glucose concentration was measured using the glucose oxidase/peroxidase technique with a commercial kit. To calculate glucose utilization, the remaining glucose in the culture medium after incubation was determined. Metformin (125 µg/mL) was used as a positive control.
Glucagon-Like Peptide-1 (GLP-1) Secretion
GLUTag cells (1 × 105 cells/well), after 24 h of incubation, were washed with KRB buffer (pH 7.2) containing HEPES (20 mM), BSA (0.2%), NaHCO3 (25.5 mM), CaCl2 (2.5 mM), MgSO4•7(H2O) (1.16 mM), KH2PO4 (1.2 mM), KCl (4.7 mM), and NaCl (0.114 M). Then, KRB buffer containing a dipeptidyl peptidase-4 inhibitor (0.2 µM), glucose (10 mM), and extract (CEL) at different concentrations were added to the cells, followed by incubation for 30 min. The total GLP-1 secretion was evaluated in the supernatants using a GLP-1 Total ELISA assay kit. Lithocholic acid (LCA; 30 µM) was used as a standard.
DPP-4 Inhibition Assay
The samples, substrate, and DPP-IV enzyme were mixed and incubated for 30 min at 37 °C. The liberated product was measured using a DPP-4 inhibitor screening assay kit (Ray Biotech, GA, USA) as per the manufacturer’s instructions.
Statistical Analysis
Statistical analysis was carried out using the SPSS/PC Version 10 package (Chicago, IL, USA). Data are expressed as mean ± SD (n = 5). The experimental data obtained from three repetitions were examined by one-way analysis of variance, followed by post hoc Duncan’s multiple range tests. P < 0.05 or less were considered statistically significant.
Results
HPLC/MS analysis was carried out to characterize the phenolic profile of the methanolic extract. The MS and UV-Vis spectra data of each chromatographic signal were compared with reference compounds and literature data, supporting the presence of 11 compounds such as Apigenin (1); Luteolin-7-galactoside (2); Aesculetin (3); Pelargonidin-3-O-glucoside (4); Peonidin-3-O-beta-galactopyranoside (5); Farnesol (6); Peonidin-3-(6″-acetylglucoside) Mesylate (7); Apigenin-C-hexoxide (8); Apigenin-O-hexosyl pentoside (9); Apiin (10); Chrysoeriol-O-hexosyl pentoside (11) (Figure 1).
Effects of CEL on INS-1 cells' viability, integrity, and mitochondria function
MTT assays were carried out to evaluate pancreatic cell viability in INS-1 cells. The addition of CEL (400, 700, 1000, 1250, and 1500 µg/mL) to pancreatic β cells for 48 h had no significant effect on cell viability (Figure 2 A). The effect on the viability of INS-1 pancreatic β-cells treated with CEL at 100, 125, 150, and 200 µg/mL together with 250 µM H2O2 for 15 min produced a decrease in cell death, with a marked increase in cell viability, with values of 53.2%, 72.3%, 77.5%, and 84.1% respectively (Figure 2 B). This finding indicates that CEL protects INS-1 cells against oxidative stress conditions.
In this study, levels of LDH were higher than those of the control group (p < 0.05). Pretreating the INS-1 cells with CEL significantly (p < 0.05) inhibited the increase of LDH levels in a dose-dependent manner (Figure 2 C). This finding indicated that CEL could inhibit the increase of LDH activity and the decrease of viability generated from H2O2 in a dose-dependent manner.
In INS-1 cells exposed to H2O2 for 2 and 4 h, there was a reduction in cellular ATP concentration when compared to the control (Figure 2 D). CEL at concentrations of 150 µg/mL and 200 µg/mL significantly (p < 0.05) increased ATP levels when compared to the control. The restoration of ATP indicates the recovery of mitochondrial membrane potential, improving the function.
Determination of insulin secretion
Low glucose treatment (5.5 mM) secreted significantly (p < 0.05) more insulin than cells treated with high glucose (30 mM) (Figure 2 E). However, treatment of INS-1 cells with glucose (30 mM) and CEL (100, 125, 150, and 200 µg/mL) increased the level of insulin secretion to 1.5-fold, 2.19-fold, and 4-fold respectively. These results were compared with GLP-I, with an increase of 5.8-fold. This finding suggests that celery treatment increases insulin secretion in INS-1 cells previously exposed to high glucose. Groups treated with H2O2 showed a significantly decreased insulin secretion of 54.44% compared to the control group. Pre-treatment with CEL prior to the addition of H2O2, insulin secretion was markedly increased by 125, 150, and 200 µg/mL treatment with 15.6%, 31.3%, and 51.0% respectively (Figure 2 F).
Hypoglycemic effect of CEL on C2C12 insulin-resistant cells
When C2C12 cells were not pretreated with palmitate and only exposed to insulin (100 nM), the inhibition of glucose production in the supernatant compared to its control group (Control) was 24%. The inhibition of glucose generation in response to insulin using C2C12 cells pretreated with palmitate resulted in a reduction of 15.4% compared with its control group (Control R), indicating that C2C12 cells present a state of resistance to insulin. Under these conditions of insulin resistance, the effect of CEL was evaluated (Figure 3 A). CEL at 150 µg/mL inhibited 82% of the glucose production in comparison with Control R. This value was greater than the percentage of inhibition obtained with the positive control, metformin (Metf 1 mM).
Activation of GLP-1 secretion and inhibition of DPP-4
The stimulatory effects of CEL on the secretion of GLP-1 were evaluated in the murine GLUTag L cell line. CEL at concentrations of 100, 125, 150, and 200 µg/mL stimulated GLP-1 secretion in GLUTag cells. A quantity of 200 µg/mL of CEL produced a significant stimulation of GLP-1 of 71.21% (Figure 3 B). The percentage of DPP-4 inhibition was determined at concentrations of celery at 100, 125, 150, and 200 µg/mL. The results are indicated in Figure 3 C, with percentage inhibition ranging from 48.16 to 82.16 µg/mL. Although CEL showed modest activity, with 48.16% inhibition at 100 µg/mL, it showed potent activity, with 82.16% inhibition at 200 µg/mL.
Discussion
In order to estimate the effect of Apium graveolens on diabetes control, we focused our study on the effects of methanolic extract in vitro models on INS-1 cells and C2C12 cell lines. Although some studies have evaluated the anti-hyperglycemic effect of celery, its mechanisms related to its antidiabetic activity remain poorly understood. Here, we characterize the mechanism of action of the hypoglycemic effect, which is mediated by the GLP-1 pathway to maximize glucose-stimulated insulin secretion.
MTT assays were carried out to evaluate pancreatic cell viability. Exposure of these INS-1 cells to a high H2O2 level (250 µM) for 48 h significantly (p < 0.05) inhibited their viability, and CEL treatment prevented this cell death. This indicates that celery protected the INS-1 cells against oxidative stress conditions.
LDH, an enzyme found in all tissues, can be used as an index of INS-1 cells damage. When INS-1 cells were exposed for a period of 24 h to a high H2O2 treatment, cytotoxicity was produced in a dose-dependent manner, together with intense membrane damage and a subsequent release of LDH.
Mitochondrial function plays an important role in the progression of type 2 diabetes due to its role in controlling cellular redox potential, oxidative phosphorylation, the release of caspase-activating proteins, ATP production, and electron transport. Interruption of these functions can lead to cell death. The preservation of mitochondrial function was examined in pancreatic cells exposed to H2O2. We have observed that CEL treatment significantly increases ATP in a dose-dependent manner, reflecting enhanced mitochondrial function and, therefore, increased cell viability with an important inhibition in the activations of death signals.
This study investigated CEL's insulin secretagogue effect in pancreatic β-cells exposed to high glucose-induced and H2O2 toxicity in pancreatic INS-1 cells as models. After administration of 125, 150, and 200 µg/mL concentrations of celery extract, we found an increased insulin secretion in pancreatic β-cells under oxidative (H2O2) and glucotoxic conditions as a result of a suppression in oxidative stress, oxidative injury, renovation of intracellular ATP, and the conservation of the morphology, function, and mitochondrial content.
In type II diabetes, its pathogenesis is characterized by β-cell dysfunction and insulin resistance. In this study, we used C2C12 cells treated with palmitate to create insulin resistance and increased reactive oxygen species (ROS) production. Previous studies have shown that metformin's capacity to enhance insulin sensitivity is related to decreased mitochondrial ROS production. Consequently, metformin was used as a standard in this experiment. CEL reduced the insulin resistance induced by palmitate in comparison with the resistance control, suggesting that CEL reverted insulin resistance similarly to metformin (metf) at the same concentration. Pancreatic β-cell mass and function can decrease during the development of type 2 diabetes, thus highlighting the necessity of searching for therapeutic agents that can preserve the mass and function of the pancreatic β-cells.
In response to the ingestion of food, the hormone glucagon-like peptide-1 (GLP-1) is secreted from enteroendocrine cells, then binds to its related receptor, and improves glucose-stimulated insulin secretion in pancreatic β-cells, ameliorating the survival of β-cells and suppressing apoptosis. In 2006, a new class of DPP-4 inhibitor was approved for the treatment of diabetic patients. DPP-4 inhibitors improve meal-stimulated insulin secretion by increasing the GLP-1 level and avoiding degradation by the enzyme DPP-4. CEL treatment, in a dose-dependent manner, was observed to significantly (p < 0.05) increase GLP-1 levels and, consequently, insulin secretion was consistent with the raised GLP-1 levels. These findings indicate that celery inhibits DPP-4 and increases GLP-1 half-lives, leading to the secretion of insulin from beta cells, which is the probable hypoglycemic mechanism of celery.
Our findings indicate that celery may protect pancreatic β-cells against glucotoxicity and H2O2 toxicity, displaying a significant effect on the recovery of mitochondrial function as a consequence of reducing oxidative stress. This leads to the diminution of apoptosis. In addition, celery increases insulin secretion and enhances insulin resistance in vitro through DPP-4 inhibition and GLP-1 activation, consequently protecting pancreatic β-cell function. This provides a possible mechanism for its antidiabetic effects. A. graveolens is a natural food that can be administered as a supplement to treat and prevent type 2 diabetes.
Conclusions
In conclusion, this research elucidated the promising hypoglycemic efficacy of Apium graveolens (celery) methanol extract through several mechanisms pertinent to diabetes management. Our findings highlighted celery's ability to enhance insulin secretion, augment pancreatic β-cell viability, and mitigate both glucotoxicity and H2O2-induced toxicity. Moreover, the inhibition of dipeptidyl peptidase-4 (DPP-4) activity by celery extract, resulting in elevated glucagon-like peptide-1 (GLP-1) levels, underscores its therapeutic potential in the regulation of glucose homeostasis. Additionally, the protective effect of celery on pancreatic β cells and its role in improving insulin sensitivity by reversing insulin resistance provide further evidence of its value in managing type 2 diabetes. These outcomes suggest that celery, rich in phenolic compounds as demonstrated through HPLC/MS analysis, offers an effective natural alternative for diabetes treatment, meriting further investigation in clinical settings. Given its accessibility and the rising interest in plant-based remedies, Apium graveolens emerges as a promising candidate for inclusion in diabetes management protocols, proposing a natural, adjunctive treatment option that complements existing therapies. This study paves the way for further research to explore the full potential of celery in diabetes care and encourages the integration of functional foods into therapeutic strategies for chronic metabolic disorders.
Abbreviations
ATP - Adenosine Triphosphate, BSA - Bovine Serum Albumin, CEL - Celery Methanol Extract, DMEM - Dulbecco’s Modified Eagle Medium, DPP-4 - Dipeptidyl Peptidase-4, ELISA - Enzyme-Linked Immunosorbent Assay, FBS - Fetal Bovine Serum, GLP-1 - Glucagon-like Peptide-1, GSIS - Glucose-stimulated Insulin Secretion, H2O2 - Hydrogen Peroxide, HEPES - 4-(2-Hydroxyethyl)-1-piperazineethanesulfonic acid, HPLC/MS - High-performance liquid chromatography / mass spectrometry, INS-1 cells - A rat pancreatic β-cell line, KRB buffer - Krebs-Ringer Buffer, LDH - Lactate Dehydrogenase, LCA - Lithocholic Acid, Metf - Metformin, MTT - 3-(4,5-Dimethylthiazol-2-yl)-2,5-diphenyltetrazolium bromide, ROS - Reactive Oxygen Species, RPMI-1640 - Roswell Park Memorial Institute Medium
Acknowledgments
None.
Author’s contributions
RMPG and JMMF carried out all studies, edited figures and revised the manuscript, checked the published data. All authors approved the final manuscript.
Funding
None.
Availability of data and materials
Data and materials used and/or analyzed during the current study are available from the corresponding author on reasonable request.
Ethics approval and consent to participate
Not applicable.
Consent for publication
Not applicable.
Competing interests
The authors declare that they have no competing interests.
References
-
Chu
Z.L.,
Jones
R.M.,
He
H.,
Carroll
C.,
Gutierrez
V.,
Lucman
A.,
A role for beta-cell-expressed G protein-coupled receptor 119 in glycemic control by enhancing glucose-dependent insulin release. Endocrinology.
2007;
148
(6)
:
2601-9
.
View Article PubMed Google Scholar -
Wajchenberg
B.L.,
beta-cell failure in diabetes and preservation by clinical treatment. Endocrine Reviews.
2007;
28
(2)
:
187-218
.
View Article PubMed Google Scholar -
Caporarello
N.,
Parrino
C.,
Trischitta
V.,
Frittitta
L.,
Insulin receptor signaling and glucagon-like peptide 1 effects on pancreatic beta cells. PLoS One.
2017;
12
(8)
:
e0181190
.
View Article PubMed Google Scholar -
Dalle
S.,
Burcelin
R.,
Gourdy
P.,
Specific actions of GLP-1 receptor agonists and DPP4 inhibitors for the treatment of pancreatic β-cell impairments in type 2 diabetes. Cellular Signalling.
2013;
25
(2)
:
570-9
.
View Article PubMed Google Scholar -
Russell-Jones
D.,
Gough
S.,
Recent advances in incretin-based therapies. Clinical Endocrinology.
2012;
77
(4)
:
489-99
.
View Article PubMed Google Scholar -
Abd El-Mageed
N.M.,
Abd
E.,
Hepatoprotective effect of feeding celery leaves mixed with chicory leaves and barley grains to hypercholesterolemic rats. Pharmacognosy Magazine.
2011;
7
(26)
:
151-6
.
View Article PubMed Google Scholar -
Ahmed
B.,
Alam
T.,
Varshney
M.,
Khan
S.A.,
Hepatoprotective activity of two plants belonging to the Apiaceae and the Euphorbiaceae family. Journal of Ethnopharmacology.
2002;
79
(3)
:
313-6
.
View Article PubMed Google Scholar -
Zidorn
C.,
Jöhrer
K.,
Ganzera
M.,
Schubert
B.,
Sigmund
E.M.,
Mader
J.,
Polyacetylenes from the Apiaceae vegetables carrot, celery, fennel, parsley, and parsnip and their cytotoxic activities. Journal of Agricultural and Food Chemistry.
2005;
53
(7)
:
2518-23
.
View Article PubMed Google Scholar -
Fazal
S.S.,
Singla
R.K.,
Review on the pharmacognostical and pharmacological characterization of Apium graveolens Linn. Indo Global Journal of Pharmaceutical Sciences.
2012;
3
(1)
:
6-42
.
View Article Google Scholar -
Perez-Gutierrez
R.M.,
V. Aguiniga Juarez,
J. Valdes Sauceda,
I. Anaya Sosa,
In vitro and in vivo antidiabetic and antiglication properties of Apium graveolens in Type 1 and 2 diabetic rats. International Journal of Pharmaceutics.
2014;
10
(7)
:
368-379
.
View Article Google Scholar -
Kooti
W.,
Ghasemiboroon
M.,
Asadi-Samani
M.,
Ahangarpoor
A.,
Zamani
M.,
Amirzargar
A.,
The effects of hydro-alcoholic extract of celery on lipid profile of rats fed a high fat diet. Advances in Environmental Biology.
2014;
68
(22)
:
325-31
.
Comments
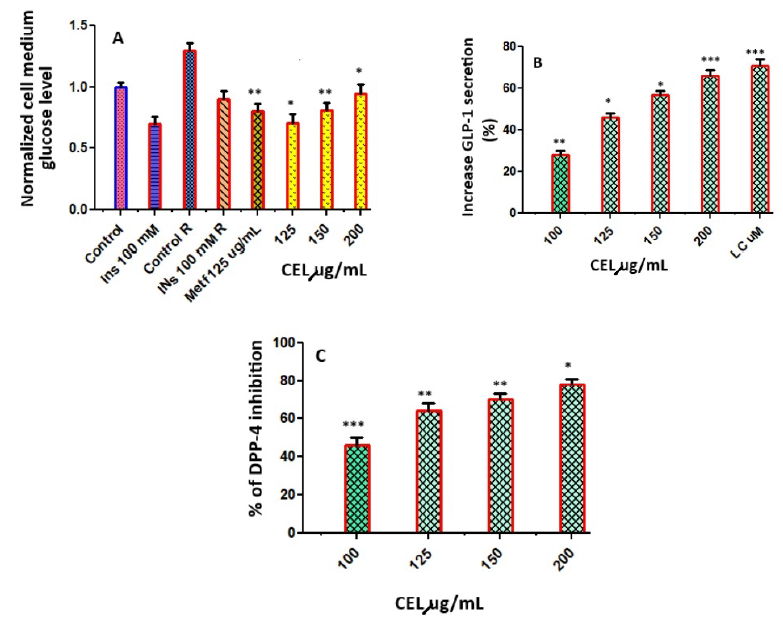
Article Details
Volume & Issue : Vol 11 No 6 (2024)
Page No.: 6548-6555
Published on: 2024-06-30
Citations
Copyrights & License

This work is licensed under a Creative Commons Attribution 4.0 International License.
Search Panel
- HTML viewed - 1383 times
- PDF downloaded - 519 times
- XML downloaded - 50 times