Abstract
Introduction: The acquisition of human mesenchymal stromal/stem cells (hMSCs) is imperative for therapeutic interventions. These versatile cells can be sourced from various fetal tissues often regarded as medical waste post-delivery. Fetal hMSCs are also procurable from aborted fetuses during the initial and early second trimesters, and amniotic fluid (hAF-MSCs) secured through amniocentesis aimed at prenatal diagnostics. This study endeavors to evaluate two economical strategies for isolating hAF-MSCs: the one-step and the two-step method, emphasizing their efficiency and potential applications in cell therapy and regenerative medicine.
Method: The comparative analysis entailed isolating hAF-MSCs using both one-step and two-step techniques. Subsequent assessment of the derived cells involved flow cytometry to detect MSC markers (CD44, CD90, and CD105) and to ascertain their capability for adipogenic and osteogenic differentiation. This methodical approach enabled an evaluation of the effectiveness of each technique in deriving a homogeneous population of hAF-MSCs suited for therapeutic applications.
Results: Examination revealed that the amniotic fluid harbors various stem/progenitor cell subpopulations characterized by distinct adhesion properties. The two-step method proved superior in deriving hAF-MSCs, especially evident in the expansion of slowly adhering amniocytes into a more uniform population of hAF-MSCs. Interestingly, prior literature scarcely addresses the adhesion characteristics of hAF-MSCs, underscoring a novel aspect of our findings.
Conclusion: This study's outcomes highlight the two-step method as a more efficacious approach for isolating hAF-MSCs, suggesting the importance of considering cell adhesion properties during isolation processes. While additional research is necessary to fully understand the efficiency of cell adhesion in the derivation of hMSCs from various sources, these initial findings pave the way for advancements in regenerative medicine and cell therapy, proposing novel considerations for optimized hMSC isolation techniques.
Introduction
Recent advancements in regenerative medicine have led to a continuous search for reliable and safe sources of stem cells with therapeutic potential. Since the first description of human mesenchymal stromal/stem cells (hMSCs) derived from bone marrow (BM)1, these cells have been considered a prominent option for cell therapy. They are easy to use in human clinical trials, banking, and cryopreservation, and possess intrinsic features such as immunosuppressive properties, low immunogenicity, homing, and differentiation capability2, 3. In addition to adult tissues like BM and adipose tissue (AT)4, hMSCs have been found in various fetal sources, including the placenta (Pl), umbilical cord (UC), and cord blood (CB), as well as in various fetal tissues such as the spleen, lung, pancreas, and kidneys5, 6, 7.
While hematopoietic stem cells (HSCs) can be accessed through an “isolation” process, obtaining hMSCs for cell therapy involves a time-consuming ex vivo expansion process known as "derivation," which varies depending on the tissue source and can impact the success of the derivation process. The source of hMSCs also plays a significant role in determining their regenerative properties8. Fetal tissues are richer in hMSCs compared to adults and can be easily obtained from normally discarded fetal and extra-fetal tissues at birth, such as the umbilical cord, cord blood, placenta, amnion, and amniotic fluid9, 10. Studies have shown that fetal sources of hMSCs have substantial advantages over adult sources in terms of regenerative capabilities. Fetal/neonatal hMSCs have been found to possess a higher anti-inflammatory and immunosuppressive capacity, advanced homing ability, and more efficient plasticity and potency, making them strong candidates for the future of regenerative medicine11, 12, 13, 14.
Amniotic fluid (AF) is an intriguing source of fetal stem cells because it is situated near various tissues in the developing fetus, including the skin, respiratory, digestive, and urogenital tracts, as well as the amnion15, 16. During the second trimester of gestation, AF is obtained through amniocentesis, a procedure commonly used in prenatal diagnosis (PND)17. The cells within the AF, known as amniocytes, comprise a diverse group of stem cells and differentiated cells derived from the three germ layers: endoderm, mesoderm, and ectoderm18. Since the amnion develops directly from the epiblast layer, it is believed that AF may contain pluripotent stem cells originating from epiblast stem cells during the pre-gastrulation period19, 20. Consequently, various clonal amniocytes can form different morphological colonies in primary and long-term cultures21, 22, 23. While some authors refer to c-Kit+ cells as amniotic fluid stem cells (AFSCs), others describe clonal amniocytes as human amniotic fluid-derived MSCs (hAF-MSCs)24, 25.
Amniotic clones exhibit various responses to long-term culture. These differences are primarily due to the cellular content of AF, the genetic background of the individuals, and the gestational age26. Additionally, the method used to isolate the AFSC populations can impact their self-renewal capacities, growth rates, and differentiation ability27. There are several methods available for isolating AFSCs, as discussed by Klemmt and colleagues27. These methods include four main cultivation techniques: two based on the propagation of enriched amniocytes pelleted by centrifugation, including one-step and two-step culture methods; the third is the starter cell culture method, which is a short-term clonal expansion approach used to isolate the fibroblastic-like cell colonies physically; and the last involves an immunosorbent approach based on surface marker selection by antigen-antibody interaction, for instance, isolation of CD117+25 and CD133+ cells28.
Previous studies have shown that the method used to isolate and culture amniocytes can significantly affect their physical characteristics and surface markers21, 27. The cost-effectiveness of the isolation method is also important, especially for animal model studies. For example, the third and fourth culture methods are not cost-effective due to the need for highly experienced personnel and expensive laboratory processes, respectively. With the presence of various subpopulations of stem/progenitor cells in the amniotic fluid exhibiting different levels of adhesion, it raises the question of whether cell adhesion influences the efficiency of hMSCs' derivation. In our research, we explored two methods for obtaining a homogeneous population of hAF-MSCs without disrupting prenatal diagnosis procedures. The two methods we studied involved using amniocytes that either quickly attach to the culture dish in a one-step process or slowly attach in a two-step process.
Methods
Culture Methods
The Ethical Committee of Yazd Reproductive Sciences Institute approved the study under the permission number "IR.SSU.REC.1396.169". Accordingly, three AF samples were collected from patients who had signed the consent form. The samples were collected from pregnant women aged 28 to 32 in their second trimester (16-18 weeks of pregnancy) for routine screening of fetal chromosomal abnormalities. The PND (prenatal diagnosis) results indicated that the samples had normal 46, XY karyotypes (male fetuses). It's important to note that high-risk pregnancies and lifestyle-related issues were excluded from the study, including tobacco, alcohol, and drug abuse, as well as pregnancy-related diseases such as history of miscarriage, gestational diabetes, and preeclampsia. We collected 16 ml of AF samples, centrifuged them at 400 g for 15 minutes, discarded the supernatant, and seeded the cell pellets in two glass Leighton tubes using AmnioMAX-II complete medium supplemented with 20 mM HEPES, 1% penicillin-streptomycin (Pen-Strep), and incubated them at 37°C and 5% CO2. The primary culture medium was refreshed on the 7th day for the first time, and then the primary cultures were subcultured after 12 to 14 days. Two days following the first passage, the cells were harvested for chromosomal analysis and concluded the PND process.
One-step method
It has been reported that amniocentesis procedures can potentially cause miscarriage (approximately 0.25%–0.50%). Therefore, it is necessary to have a valid medical reason for collecting human amniocytes, and the use of these cells for research should not interfere with the prenatal diagnostic process. In our case, the remaining Leighton tubes from the diagnostic process were utilized to derive hAF-MSCs using a one-step method. For this, the cells were cultured sequentially at a density of 104 cells/cm2 in a T25 flask and incubated at 37°C and 5% CO2. For this purpose, a modified medium composed of 2:1 v/v DMEM/AmnioMAX-II was used since our previous study had shown its higher efficiency compared to the DMEM and AmnioMAX-II alone21. The DMEM was supplemented with 4 mM L-glutamine, 10 mM HEPES, 15% fetal bovine serum (FBS), and 1% Pen-Strep (all from Gibco).
Two-step method
Human amniocytes have varying adhesive properties, making it difficult for many cells to attach to the surface of culture dishes during primary cultivation. The non-adherent cells, which are typically discarded during the first medium-refreshing step on the 7th day of cultivation, were utilized to derive hAF-MSCs through a two-step method without interfering with the PND process. However, the number of these cells is generally limited because they are removed from the primary culture without undergoing cell expansion (1-3 × 105 cells in each glass Leighton tube). They were collected by centrifugation, then seeded at a density of 103 cells/cm2 into a 6-well plate (about 104 cells per well) using a modified DMEM-AmnioMAX-II medium (2:1 v/v) supplemented in the same way as the one-step method. The clones from each well were seeded at a density of 104 cells/cm2 in a T25 flask using the same modified medium. The cell expansion process was common to both methods and continued until the third passage when the cells were harvested for flow cytometry analysis and culturing under differentiation conditions. The cells were subcultured at a confluence of 70-80% by washing with phosphate-buffered saline (PBS), treated with 0.05% Trypsin-EDTA (Gibco), and deactivated with the same volume of modified medium. The cultures were incubated at 37°C and under 5% humidified CO2 in both methods. A detailed schematic illustration of the culture methods can be found in Figure 1.
Molecular analysis
To assess the pluripotency of cells isolated by two different methods, we conducted a qualitative analysis using RT-PCR to examine a panel of stemness markers, including OCT4, NANOG, SOX2, C-KIT (CD117), C-MYC, and THY1 (CD90). The thermal cycling for gene amplification was carried out in Applied Biosystems thermal cyclers (VeritiPro™) with the following protocol: an initial holding stage at 94°C for 5 minutes, followed by 35 cycles of 94°C for 20 seconds, 58°C for 30 seconds, and 72°C for 1 minute. The final stage involved holding at 72°C for 7 minutes. Subsequently, the PCR products were separated on a 2% agarose gel stained with a fluorescent dye (DNA Green Viewer) and visualized using a UV Gel Doc system. Additionally, we used RT-PCR to quantitatively assess the expression of specific differentiation markers, such as peroxisome proliferator-activated receptor gamma (PPARγ) for adipogenic differentiation and runt-related transcription factor 2 (RUNX2) for osteogenic differentiation. The 18S rRNA served as the reference gene for quantitative analysis. To achieve this, we isolated total RNA from the cells cultured under differentiation conditions (after 21 days) using the Qiagen RNeasy™ Mini Kit according to the manufacturer's manual. Next, the extracted RNA (normalized to 200ng) was utilized to synthesize cDNA with the RevertAid First Strand cDNA Synthesis Kit (Thermo Scientific) following the manufacturer's protocols. Quantitative RT-PCR analysis was performed using the Applied Biosystems StepOnePlus Real-Time PCR System, which involved an initial denaturation step at 95°C for 10 minutes, followed by 40 cycles of 95°C for 10 seconds and 60°C for 40 seconds. We employed the Applied Biosystems® SYBR® Green PCR Master Mix for the PCR reactions, and human-specific, intron-spanning primers were designed for the gene targets listed in Table 1.
Gene | Primer | Product Size (base pairs) |
---|---|---|
OCT4 | Forward: GATGTGGTCCGAGTGTGGTT | 245 bp |
Reverse: AGAGTGGTGACGGAGACAGG | ||
NANOG | Forward: TTTGGAAGCTGCTGGGGAAG | 194 bp |
Reverse: GATGGGAGGAGGGGAGAGGA | ||
SOX2 | Forward: GCCGAGTGGAAACTTTTGTCG | 154 bp |
Reverse: GCAGCGTGTACTTATCCTTCTT | ||
C-MYC | Forward: TGGTCGCCCTCCTATGTTG | 151 bp |
Reverse: CCGGGTCGCAGATGAAACTC | ||
C-KIT | Forward: CCAACACCGGCAAATACACG | 250 bp |
Reverse: TTGATCATGATGCCCGCCTT | ||
THY1 | Forward: TCAGCATCGCTCTCCTGCTA | 120 bp |
Reverse: TGCTGGTATTCTCATGGCGG | ||
PPARγ | Forward: TTATTCTCAGTGGAGACCGCC | 110 bp |
Reverse: CTCAGGGTGGTTCAGCTTCA | ||
RUNX2 | Forward: GCGCATTCCTCATCCCAGTAT | 120 bp |
Reverse: TGCCTGGGGTCTGTAATCTG | ||
18s rRNA | Forward: AGAAACGGCTACCACATCCA | 158 bp |
Reverse: CCCTCCAATGGATCCTCGTT |
Flow cytometry analysis
The characterization of the cell isolates was performed using flow cytometry by examining three well-known MSC markers CD44, CD90, and CD105. We also used the hematopoietic/endothelial marker CD31 as a negative marker for analysis. Simultaneously with the differentiation assay, we harvested the cells through centrifugation and rinsed them twice in PBS with 0.2% FBS. Following this, the cells were treated with designated conjugated antibodies in a PBS solution supplemented with 1% BSA: fluorescein isothiocyanate (FITC)-CD44 (from Immunostep), FITC-CD90, phycoerythrin (PE)-CD105 (both from Exbio), and PE-CD31 (from Immunostep). Finally, we analyzed the cells using a BD FACSCalibur and generated the graphics in the FlowJo (v 10.1, Tree Star, Inc.) software.
Differentiation capacity
We conducted an investigation to determine if isolated cells could be converted into adipogenic and osteogenic lineages. For this purpose, the cells were allowed to grow until the third passage, reaching 80% confluency, and then were placed under differentiation conditions for 21 days. The medium was changed every 3 days. The adipogenic medium consisted of DMEM supplemented with 10% FBS, 1% Penicillin-Streptomycin, 50 μg/ml indomethacin, 50 μg/ml ascorbic acid-2-phosphate, 10 IU/ml insulin, and 100 nM dexamethasone. The osteogenic medium contained DMEM supplemented with 10% FBS, 1% Penicillin-Streptomycin, 10 mM β-glycerophosphate, 50 μg/ml ascorbic acid-2-phosphate, and 10 nM dexamethasone, all from Sigma-Aldrich. To confirm adipogenesis, the cells were stained with Oil Red O (Sigma-Aldrich) to verify the presence of intracellular lipid vacuoles. After washing with PBS, the cells were fixed in 4% paraformaldehyde for 45 minutes and then incubated with 60% isopropanol for 5 minutes, both at room temperature. For staining, the cells were first incubated with Oil Red O and then counterstained with hematoxylin for 15 and 1 minute, respectively. Osteogenesis validation was observed by staining the extracellular matrix mineralization with Alizarin Red. Similarly, the cells were washed with PBS and fixed in 4% paraformaldehyde for 10 minutes. For staining, the cells were first washed with distilled water, then covered with a 2% Alizarin Red solution for 10 minutes, and finally washed with distilled water three times29.
Statistical analysis
The RT-PCR results were quantitatively assessed using the 2ΔCT methods, which take into account the difference between reference and target CT values for analysis. To compare the average expression levels across three samples, a non-parametric t-test was performed with a significance level at a p-value < 0.05. Additionally, the lifespan of cells was calculated by determining the mean value of passages during both long-term cultures, and a comparison between the two methods was made using a non-parametric t-test (p-value < 0.05). Statistical analysis and illustration of plots were carried out using GraphPad Prism 8 software.
Results
Outcome of Culture Methods on Primary and Expanded Clones
Our daily microscopic observations unveiled a striking difference between the two culture methods, leading to the identification of various morphological clones, including epithelioid and fibroblastoid cells, in the primary cultures established through the one-step process, namely in glass Leighton tubes as part of the PND procedure (Figure 2 A). However, only fibroblastoid clones emerged in the two-step primary cultures in 6-well plates, which were developed from non-adherent amniocytes collected during the initial medium refreshment on the 7th day of primary cultures in Leighton tubes considered as discarded leftover from the PND procedure (Figure 2 B). Interestingly, during the three-passaged culture of the one-step method, two out of three samples displayed visible three-dimensional (3D) structures without the need for magnification, as shown in Figure 2 C and D, whereas none were observed in the two-step method.In the third passage, noticeable differences in the morphology of cell populations were observed between the two methods. Specifically, when three samples were cultured using the one-step method, they contained various cell populations with different appearances, including epithelioid and fibroblastoid cells, and even cells displaying characteristics of epithelial to mesenchymal transition (EMT) (Figure 2 E). On the other hand, when the same samples were expanded through the two-step method, the result was a homogeneous population of fibroblastoid cells at the end of the third passage (Figure 2 F). Beyond the third passage, the experiment continued to determine the point at which cells begin to lose their ability to proliferate, a process known as replicative senescence. The results, based on the average passage numbers, showed that the samples cultured using the two-step method had a longer lifespan (8.3 passages) compared to the samples cultured using the one-step method, which had a lower value (4.3 passages) (p-value < 0.05).
Expression of Stemness Markers in Expanded Clones from Two Methods
To compare the stemness of cells obtained by the two methods, we assessed the expression of stem cell markers, focusing on the core regulatory circuit of human embryonic stem cells (hESCs): OCT4, NANOG, and SOX2. Our findings indicated that these genes were expressed in the clones obtained through the one-step method. Conversely, SOX2 and OCT4 were found to be unexpressed in clones S1 and S2, respectively, obtained through the two-step method. Additionally, the expression of NANOG was significantly lower in S2 compared to the other two samples. We also observed that the C-KIT expression was absent in S1 and S2 expanded through the one-step and two-step methods, respectively. As illustrated in Figure 3 A, it is evident that the THY1 gene was expressed at a low level in S1 obtained through the one-step method.
MSC-Specific Markers in Expanded Clones from Two Methods
The flow cytometry analysis was used to characterize the expanded clones using two methods based on MSC-specific markers. The results, shown in Figure 4 and Figure 5, indicated that over 95% of the cells from both methods were negative for CD31, suggesting their non-hematopoietic/endothelial origin. However, the analysis of markers CD44, CD90, and CD105 in the cells from the two methods revealed that the clones expanded using the one-step method were highly diverse, with only a fraction of the cells expressing the desired markers. In contrast, the cells from the two-step method formed a homogeneous population, with over 95% of the cells expressing the desired markers. A statistical comparison of the percentage of cells expressing the desired markers showed that the two-step method significantly expressed MSC markers at a higher level compared to the one-step method (p-value < 0.05) (Figure 3 B).
Differentiation Capabilities of Clones from Two Culture Methods
The differentiation evaluation results showed that cells obtained from both methods were able to differentiate into adipocytes and osteocytes. As shown in panel A of Figure 6, cells from both the one-step and two-step methods exhibited intracellular lipid vacuoles, a typical characteristic of mature adipocytes. Microscopic evaluation of cultures under osteogenic conditions also showed similar results, with all samples from both groups displaying calcium deposits on their surface (panel B: Figure 6 A-F). However, quantitative RT-PCR analysis revealed a significant difference between the methods. The results indicated that the adipogenic marker PPARγ was expressed nearly two-fold higher in the two-step compared to the one-step culture method (p-value < 0.05). Similarly, the analysis of RUNX2, a well-known osteogenic marker, showed a non-significant higher level in the cells derived from the two-step method (p-value < 0.05) (Figure 3 C).
Discussion
Amniocytes released into the amniotic fluid (AF) can range from fully differentiated and lineage-committed cells to pluripotent and highly multipotent stem cells capable of developing into various cell types18. These amniocytes comprise a highly diverse population with distinct biochemical, morphological, and growth characteristics21, 30. Previous research has shown that long-term culture of human AF has led to the expansion of highly clonogenic cell types, including epithelioid and fibroblast-like cells21, 22. However, it is unclear whether these cells can be classified as stem cells. Our previous work on deriving hAF-MSCs led us to this study, as we found that the culture protocol significantly influences the successful derivation of these cells. For example, in our research involving 68 samples of human amniocytes cultured using a one-step method, we found that only 70% of long-term cultures resulted in the isolation of homogeneous cell populations, comprising various epithelioid and fibroblast-like cells21, 23. In contrast, a two-step method demonstrated higher efficiency (more than 85%) in achieving a homogeneous fibroblast cell population during long-term cultures26. Based on these prior findings, we designed the current study to objectively compare two culture methods and their performance in deriving hAF-MSCs.
It is essential to carefully consider the method used to obtain hMSCs based on the tissue source, as it can affect the regenerative characteristics of hMSCs8. Based on studies that have examined fetal and adult MSCs from different aspects of regeneration, fetal sources of MSCs seem to have substantial advantages over adult MSCs18, 31. Fetal tissues contain a higher concentration of hMSCs compared to adults. It has been demonstrated that hMSCs constitute a small portion of the cell population in tissues that are more abundant during fetal life than in adulthood. For example, in the second trimester of pregnancy, hMSCs account for about 1 in 3000 blood cells and 1 in 400 bone marrow cells. However, in a healthy newborn and an 80-year-old individual, these ratios drop to 1 in 10,000 and 1 in 2 million cells, respectively32, 33. Moreover, experimental studies have shown that fetal/neonatal hMSCs have more potent therapeutic properties compared to adult/somatic MSCs, including higher anti-inflammatory and immunosuppressive capacities, improved homing ability, and notably greater plasticity and potency11, 12, 13. Therefore, these characteristics make fetal hMSCs promising candidates for the development of future regenerative medicine strategies.
Various methods have been suggested for deriving hMSCs from fetal and adult tissues. Most of these methods rely on the adhesion property of hMSCs for their primary isolation from the tissue source. For example, in some methods of deriving hAF-MSCs, the cells are first passed through a 40-micrometer (µm) cell strainer to remove tissue debris and subsequently collected from the amniotic fluid (AF) by centrifugation. The cell pellet is then cultured during an in vitro cell expansion process to derive hAF-MSCs. The culture medium used in this method is α-MEM medium supplemented with 18% Chang B and 2% Chang C media34, 35. Another commonly used technique involves immunoselection targeting the CD117 (c-KIT) tyrosine kinase receptor, pioneered by De Coppi et al. to isolate CD117⁺ AF-MSCs from mice25. This approach entails the initial adhesion of amniocytes to culture dishes and their proliferation until reaching 70% confluency. Subsequently, CD117-based immunoselection is performed using magnetic microbeads tagged with its ligand, followed by a sequential in vitro cell expansion process to finally derive CD117⁺ cells25, 34, 35. A mechanical isolation procedure, known as the “starter cell” method, is also used to derive AF-MSCs27, 35, 36. Initially, amniocytes are obtained from AF and filtered through a 40-µm strainer. The collected cells are then plated in a culture dish. When the first attached cells appear in the primary culture, they are observed for several days until individual single starter cells form colonies. To prevent confluence with neighboring colonies, cells that grow too closely together are removed manually. Using an inverted microscope and fine-tipped pipettes, each colony is mechanically isolated. Cells aspirated from each colony are then transferred into individual wells until they reach 70% confluency, following which they are sequentially sub-cultured onto plates with larger surface areas36.
Several methods have been developed to isolate MSCs from adult sources such as bone marrow and adipose tissue. Ex vivo-expanded MSCs from these sources have shown consistent characteristics in terms of viability, morphology, proliferation rate, surface marker expression, cytokine secretion levels, and differentiation capacity37. However, our previous studies on different clones of hAF-MSCs have revealed significant differences in morphology, proliferation rate, lifespan, and expression of stemness and immunomodulatory marker genes21, 22, 23. Some findings suggest that the outcome of the culture method depends on the adhesive properties of amniocytes, which can be influenced by culture conditions, such as medium, supplements, culture dish surfaces, and interactions with other cells27. Our current study employed two adhesion-based approaches to derive clonal amniocytes, comparing their efficiency in achieving hAF-MSCs. The one-step method yielded fast-adherent cells with strong adhesion properties but greater heterogeneity following long-term culture. The non-adherent cells were harvested by refreshing the medium on the 7th day of primary culture as the cell source of the two-step method. As a result, the slowly adherent amniocytes are considered to lead to a more homogeneous population of cells that express the desired MSC markers, referred to as hAF-MSCs.
It is important to note that using the supernatant from a 7-day primary culture may not be the optimal time for isolating non-adherent cells as the cell source in the two-step method. Additionally, obtaining human amniocytes for research purposes raises ethical concerns due to the risk of miscarriage associated with amniocentesis. Therefore, one of the main objectives of this study was to ensure that the cultivation methods align with the clinical process of PND to obtain hAF-MSCs with minimal interference. Thus, we utilized the discarded medium from the 7-day primary culture. The two-step method, established by Tsai and colleagues in 2004, achieved the derivation of hAF-MSCs by directly seeding human amniocytes from the primary culture's supernatant into T25 flasks38. However, our pilot study using this approach did not yield significantly better results than the one-step method. As a result, we adapted the method by first seeding the cells from the supernatant of the primary culture onto a 6-well plate and then expanding them in a T25 flask. In addition, MSC marker analysis by flow cytometry shows that the two-step method is more effective than the one-step method in deriving AF-MSCs. Moreover, RT-PCR analysis of the adipocyte marker PPARγ suggests heightened differentiation efficiency in cells derived using the two-step method.
In the past ten years, various three-dimensional (3D) cell culture methods have been developed, enabling the study of cell-cell or cell-extracellular matrix (ECM) interactions in a microenvironment that more closely mimics physiological conditions. These methods are crucial for researching embryonic development, tumor-stromal cell interactions in cancer invasion, wound healing, and tissue engineering39. Research has shown that spheroid aggregates of MSCs have significant impacts on their signaling and function, particularly in stemness and immune modulation40, 41, 42, 43. Although research on hMSCs continues, understanding their potential for transformation and tumorigenicity remains a gap in cell therapy approaches44. On the other hand, while undifferentiated hESCs have the potential for treating disorders, they may form benign or malignant masses in vivo, known as teratomas and embryonic carcinomas, respectively, possibly hindering future therapies45. Unlike hESCs, hMSCs become senescent after long-term expansion, which reduces their tumorigenic potential. Therefore, there has been a growing interest in hMSCs due to the absence of concerns associated with hESCs11, 12, 13.
Pluripotent stem cells (PSCs) are known for their ability to form spheroid structures in vitro, which depend on the activation of the nuclear pluripotency circuit, namely OCT4, NANOG, and SOX246. Many reports have suggested that AF-MSCs possess intermediate characteristics related to the expression of stem cell markers associated with pluripotency and multipotency identity13, 47. The isolation of a subpopulation of human amniocytes that express OCT4 and C-KIT by Lee and colleagues suggests the possible presence of PSCs or cells in a developmental stage that can easily convert into pluripotent cells48. The study by Moschidou et al. in 2012 found that culturing CD117+ human amniotic fluid-derived MSCs on Matrigel in an hESC medium supplemented with valproic acid, a histone deacetylase inhibitor, reprogrammed these cells into a pluripotent state with more than 80% similarity in expression profile to hESCs and the ability to form embryoid bodies in vitro and teratomas in vivo49. Additionally, Ditadi and colleagues have demonstrated that CD117+ AFSCs exhibit hematopoietic stem cell markers and the capacity for myeloid and erythroid differentiation50. In conclusion, some studies have shown that AF-derived stem cells (AFSCs) can express both pluripotency and MSC markers simultaneously13, 47, 51, 52, while other reports have confirmed the isolation of AF-MSCs with only specific MSC markers such as CD44, CD73, CD90, and CD10538, 53, 54, 55, 56. These findings are consistent with the idea that the amniotic cavity serves as a cellular reservoir containing various cell subpopulations released by different extra-embryonic membranes and fetal tissues into the amniotic fluid.
It has been suggested that human amniotic epithelial cells (hAECs) released by the amnion into the amniotic fluid may maintain characteristics of pluripotency similar to epiblast stem cells30, 47. As the amnion is separated from the epiblast during early embryo development, epiblast stem cells may not receive signals prompting differentiation into the germ layers, i.e., ectoderm, mesoderm, and endoderm15. Our study on the nuclear pluripotency circuit revealed that all three samples cultured using the one-step method fully expressed OCT4, NANOG, and SOX2 genes. This may be due to the presence of epithelioid cells and the heterogeneity of cultures from the one-step method. Spheroid structures, reported in primary cultures of human amniocytes as self-generating structures21, were also observed in long-term cultures from the one-step method. Nonetheless, the absence of spheroid structures in clones expanded via the two-step method may be attributed to their dependency on interactions with other cell types present in the heterogeneous cultures of the one-step method. Additionally, our study indicated that homogeneous fibroblastic clones from the two-step method did not fully express the nuclear pluripotency circuit genes. Our previous research showed that the key markers of pluripotency were significantly more expressed in epithelioid clones compared to fibroblastic clones, suggesting a closer similarity in expression profile between epithelioid clones and hESCs22, which could account for the generation of spherical structures during long-term cultures in the one-step method.
Conclusions
Deriving stem cells from human amniocytes has involved several established methods, some of which necessitate substantial practical skills or involve costly techniques, such as physically isolating clones or employing immunoabsorbent approaches based on surface marker selection. In this study, we used two sequential subculturing-based methods, and the results imply differences in efficiency and cell characteristics between the two methods. Besides their different morphological appearances, the flow cytometry results showed that the two-step method yielded homogeneous clones, whereas the one-step method resulted in a heterogeneous population of fibroblastic and epithelioid clones. It has been suggested that epithelioid clones might have originated from hAECs with the ability to express nuclear pluripotency circuit genes (OCT4, NANOG, and SOX2). Our findings demonstrated that all three long-term cultures obtained using the one-step method expressed these key pluripotency markers. Additionally, the presence of spheroid structures during long-term cultures of the one-step method, which is believed to form due to interactions with other cell types, especially PSCs, further reinforces the idea of the heterogeneous nature of one-step cultures. According to our findings, the primary culture of human amniocytes as the source of hAF-MSCs plays a crucial role in the successful derivation process. As a result, the slowly adherent amniocytes collected through the two-step method may have more potential to generate a homogeneous population of fibroblastic clones expressing the desired MSC markers, referred to as hAF-MSCs.
Abbreviations
3D - Three-Dimensional, AF - Amniotic Fluid, AFSCs - Amniotic Fluid Stem Cells, AT - Adipose Tissue, BM - Bone Marrow, CB - Cord Blood, DMEM - Dulbecco's Modified Eagle Medium, EMT - Epithelial to Mesenchymal Transition, FBS - Fetal Bovine Serum, FITC - Fluorescein Isothiocyanateh, AECs - Human Amniotic Epithelial Cellsh, AF-MSCs - Human Amniotic Fluid-derived Mesenchymal Stem Cells, hESCs - Human Embryonic Stem Cells, HSCs - Hematopoietic Stem Cells, hMSCs - Human Mesenchymal Stromal/Stem Cells, MSC - Mesenchymal Stem Cell, PBS - Phosphate-Buffered Saline, Pen-Strep - Penicillin-Streptomycin, PE - Phycoerythrin, Pl - Placenta, PND - Prenatal Diagnosis, PPARγ - Peroxisome Proliferator-Activated Receptor Gamma, PSCs - Pluripotent Stem Cells, RT-PCR - Reverse Transcription Polymerase Chain Reaction, RUNX2 - Runt-Related Transcription Factor 2, UC - Umbilical Cord
Acknowledgments
This study was funded by Shahid Sadoughi University of Medical Sciences and Health Services. The work was carried out within the framework of a project (code: 5664) sponsored by the Abortion Research Center, Yazd Reproductive Sciences Institute. We also gratefully acknowledge Zeynab Darvishian and Sama Ghasemi for their technical assistance in flow cytometry analysis.
Author’s contributions
All authors equally contributed to this work, read and approved the final manuscript.
Funding
This study was funded by Shahid Sadoughi University of Medical Sciences and Health Services. The work was carried out within the framework of a project (code: 5664) sponsored by the Abortion Research Center, Yazd Reproductive Sciences Institute.
Availability of data and materials
Data and materials used and/or analyzed during the current study are available from the corresponding author on reasonable request.
Ethics approval and consent to participate
The study was approved by the Ethical Committee of Yazd Reproductive Sciences Institute, Shahid Sadoughi University of Medical Sciences, under the permission number “IR.SSU.REC.1396.169”. The samples were collected from patients who had signed a written consent form to allow their surplus cells to be used in research.
Consent for publication
Not applicable.
Competing interests
The authors declare that they have no competing interests.
References
-
Pittenger
M.F.,
Mackay
A.M.,
Beck
S.C.,
Jaiswal
R.K.,
Douglas
R.,
Mosca
J.D.,
Multilineage potential of adult human mesenchymal stem cells. Science.
1999;
284
(5411)
:
143-7
.
View Article PubMed Google Scholar -
Ullah
I.,
Subbarao
R.B.,
Rho
G.J.,
Human mesenchymal stem cells - current trends and future prospective. Bioscience Reports.
2015;
35
(2)
:
e00191
.
View Article PubMed Google Scholar -
Hoseini
S.,
Montazeri
F.,
Kalantar
S.,
Bahrami
A.,
Zarein
F.,
Moghadam matin M. Mesenchymal Stem Cells: Interactions with Immune Cells and Immunosuppressive-Immunomodulatory Properties. The Scientific Journal of Iranian Blood Transfusion Organization..
2020;
17
(2)
:
147-69
.
-
Kern
S.,
Eichler
H.,
Stoeve
J.,
Klüter
H.,
Bieback
K.,
Comparative analysis of mesenchymal stem cells from bone marrow, umbilical cord blood, or adipose tissue. Stem Cells (Dayton, Ohio).
2006;
24
(5)
:
1294-301
.
View Article PubMed Google Scholar -
in 't Anker
P.S.,
Noort
W.A.,
Scherjon
S.A.,
Kleijburg-van der Keur
C.,
Kruisselbrink
A.B.,
van Bezooijen
R.L.,
Mesenchymal stem cells in human second-trimester bone marrow, liver, lung, and spleen exhibit a similar immunophenotype but a heterogeneous multilineage differentiation potential. Haematologica.
2003;
88
(8)
:
845-52
.
PubMed Google Scholar -
In 't Anker
P.S.,
Scherjon
S.A.,
Kleijburg-van der Keur
C.,
de Groot-Swings
G.M.,
Claas
F.H.,
Fibbe
W.E.,
Isolation of mesenchymal stem cells of fetal or maternal origin from human placenta. Stem Cells (Dayton, Ohio).
2004;
22
(7)
:
1338-45
.
View Article PubMed Google Scholar -
Hu
Y.,
Liao
L.,
Wang
Q.,
Ma
L.,
Ma
G.,
Jiang
X.,
Isolation and identification of mesenchymal stem cells from human fetal pancreas. The Journal of Laboratory and Clinical Medicine.
2003;
141
(5)
:
342-9
.
View Article PubMed Google Scholar -
Hoseini
S.,
Hosseini
E.S.,
Abessi
P.,
Montazeri
F.,
Paracrine secretions and immunological activities of human mesenchymal stem cells; the key regenerative factors of microenvironment. Journal of Stem Cell Research & Therapy.
2024;
14
(1)
:
636
.
-
Ullah
I.,
Subbarao
R.B.,
Rho
G.J.,
Human mesenchymal stem cells - current trends and future prospective. Bioscience Reports.
2015;
35
(2)
:
e00191
.
View Article PubMed Google Scholar -
Sousa
B.R.,
Parreira
R.C.,
Fonseca
E.A.,
Amaya
M.J.,
Tonelli
F.M.,
Lacerda
S.M.,
Human adult stem cells from diverse origins: an overview from multiparametric immunophenotyping to clinical applications. Cytometry. Part A.
2014;
85
(1)
:
43-77
.
View Article PubMed Google Scholar -
Antonucci
I.,
Pantalone
A.,
Tete
S.,
Salini
V.,
Borlongan
C.V.,
Hess
D.,
Amniotic fluid stem cells: a promising therapeutic resource for cell-based regenerative therapy. Current Pharmaceutical Design.
2012;
18
(13)
:
1846-63
.
View Article PubMed Google Scholar -
Di Trapani
M.,
Bassi
G.,
Fontana
E.,
Giacomello
L.,
Pozzobon
M.,
Guillot
P.V.,
Immune regulatory properties of CD117(pos) amniotic fluid stem cells vary according to gestational age. Stem Cells and Development.
2015;
24
(1)
:
132-43
.
View Article PubMed Google Scholar -
Loukogeorgakis
S.P.,
De Coppi
P.,
Concise Review: Amniotic Fluid Stem Cells: The Known, the Unknown, and Potential Regenerative Medicine Applications. Stem Cells (Dayton, Ohio).
2017;
35
(7)
:
1663-73
.
View Article PubMed Google Scholar -
Sagar
R.,
Walther-Jallow
L.,
David
A.L.,
Götherström
C.,
Westgren
M.,
Fetal Mesenchymal Stromal Cells: an Opportunity for Prenatal Cellular Therapy. Current Stem Cell Reports.
2018;
4
(1)
:
61-8
.
View Article PubMed Google Scholar -
Dobreva
M.P.,
Pereira
P.N.,
Deprest
J.,
Zwijsen
A.,
On the origin of amniotic stem cells: of mice and men. The International Journal of Developmental Biology.
2010;
54
(5)
:
761-77
.
View Article PubMed Google Scholar -
Gosden
C.M.,
Amniotic fluid cell types and culture. British Medical Bulletin.
1983;
39
(4)
:
348-54
.
View Article PubMed Google Scholar -
Mather
J.P.,
Stem cell culture. Preface. Methods in Cell Biology.
2008;
86
:
xv-xvii
.
View Article PubMed Google Scholar -
Hoseini
S.M.,
Moghaddam-Matin
M.,
Bahrami
A.R.,
Montazeri
F.,
Kalantar
S.M.,
Human Amniotic Fluid Stem Cells: General Characteristics and Potential Therapeutic Applications. The Journal of Shahid Sadoughi University of Medical Sciences..
2021;
28
(12)
:
3252-75
.
View Article Google Scholar -
Pappa
K.I.,
Anagnou
N.P.,
Novel sources of fetal stem cells: where do they fit on the developmental continuum?. Regenerative Medicine.
2009;
4
(3)
:
423-33
.
View Article PubMed Google Scholar -
Ilancheran
S.,
Michalska
A.,
Peh
G.,
Wallace
E.M.,
Pera
M.,
Manuelpillai
U.,
Stem cells derived from human fetal membranes display multilineage differentiation potential. Biology of Reproduction.
2007;
77
(3)
:
577-88
.
View Article PubMed Google Scholar -
Hoseini
S.M.,
Kalantar
S.M.,
Bahrami
A.R.,
Matin
M.M.,
Human Amniocytes: a Comprehensive Study on Morphology, Frequency and Growth Properties of Subpopulations from a Single Clone to the Senescence. Cell and Tissue Biology.
2020;
14
(2)
:
102-12
.
View Article Google Scholar -
Hoseini
S.M.,
Montazeri
F.,
Bahrami
A.R.,
Kalantar
S.M.,
Rahmani
S.,
Zarein
F.,
Investigating the expression of pluripotency-related genes in human amniotic fluid cells: A semi-quantitative comparison between different subpopulations, from primary to cultured amniocytes. Reproductive Biology.
2020;
20
(3)
:
338-47
.
View Article PubMed Google Scholar -
Hoseini
S.M.,
Sheikhha
M.H.,
Kalantar
S.M.,
Matin
M.M.,
Aflatoonian
B.,
Bahrami
A.R.,
A comparative analysis of immunomodulatory genes in two clonal subpopulations of CD90+ amniocytes isolated from human amniotic fluid. Placenta.
2020;
101
:
234-41
.
View Article PubMed Google Scholar -
Maguire
C.T.,
Demarest
B.L.,
Hill
J.T.,
Palmer
J.D.,
Brothman
A.R.,
Yost
H.J.,
Genome-wide analysis reveals the unique stem cell identity of human amniocytes. PLoS One.
2013;
8
(1)
:
e53372
.
View Article PubMed Google Scholar -
De Coppi
P.,
Bartsch
G.,
Siddiqui
M.M.,
Xu
T.,
Santos
C.C.,
Perin
L.,
Isolation of amniotic stem cell lines with potential for therapy. Nature Biotechnology.
2007;
25
(1)
:
100-6
.
View Article PubMed Google Scholar -
F. Montazeri,
M. Tajamolian,
E.S. Hosseini,
S.M. Hoseini,
Immunologic Factors and Genomic Considerations in Recurrent Pregnancy Loss: A Review. International Journal of Medical Laboratory.
2023;
10
(4)
:
279 -305
.
View Article Google Scholar -
Klemmt
P.A.,
Vafaizadeh
V.,
Groner
B.,
The potential of amniotic fluid stem cells for cellular therapy and tissue engineering. Expert Opinion on Biological Therapy.
2011;
11
(10)
:
1297-314
.
View Article PubMed Google Scholar -
Schmidt
D.,
Achermann
J.,
Odermatt
B.,
Breymann
C.,
Mol
A.,
Genoni
M.,
Prenatally fabricated autologous human living heart valves based on amniotic fluid derived progenitor cells as single cell source. Circulation.
2007;
116
(11)
:
64-70
.
View Article PubMed Google Scholar -
Kornicka
K.,
Marycz
K.,
Tomaszewski
K.A.,
Marędziak
M.,
Śmieszek
A.,
The effect of age on osteogenic and adipogenic differentiation potential of human adipose derived stromal stem cells (hASCs) and the impact of stress factors in the course of the differentiation process. Oxidative medicine and cellular longevity.
2015;
2015
(1)
:
309169
.
View Article Google Scholar -
Simoni
G.,
Colognato
R.,
The amniotic fluid-derived cells: the biomedical challenge for the third millennium. Journal of Prenatal Medicine.
2009;
3
(3)
:
34-6
.
PubMed Google Scholar -
Yin
J.Q.,
Zhu
J.,
Ankrum
J.A.,
Manufacturing of primed mesenchymal stromal cells for therapy. Nature Biomedical Engineering.
2019;
3
(2)
:
90-104
.
View Article PubMed Google Scholar -
Caplan
A.I.,
The mesengenic process. Clinics in Plastic Surgery.
1994;
21
(3)
:
429-35
.
View Article PubMed Google Scholar -
Sagar
R.,
Walther-Jallow
L.,
David
A.L.,
Götherström
C.,
Westgren
M.,
Fetal Mesenchymal Stromal Cells: an Opportunity for Prenatal Cellular Therapy. Current Stem Cell Reports.
2018;
4
(1)
:
61-8
.
View Article PubMed Google Scholar -
Zia
S.,
Toelen
J.,
Mori da Cunha
M.,
Dekoninck
P.,
de Coppi
P.,
Deprest
J.,
Routine clonal expansion of mesenchymal stem cells derived from amniotic fluid for perinatal applications. Prenatal Diagnosis.
2013;
33
(10)
:
921-8
.
View Article PubMed Google Scholar -
DeKoninck
P.,
Toelen
J.,
Zia
S.,
Albersen
M.,
Lories
R.,
Coppi
P.D.,
Routine isolation and expansion late mid trimester amniotic fluid derived mesenchymal stem cells in a cohort of fetuses with congenital diaphragmatic hernia. European Journal of Obstetrics, Gynecology, and Reproductive Biology.
2014;
178
:
157-62
.
View Article PubMed Google Scholar -
Roubelakis
M.G.,
Bitsika
V.,
Zagoura
D.,
Trohatou
O.,
Pappa
K.I.,
Makridakis
M.,
In vitro and in vivo properties of distinct populations of amniotic fluid mesenchymal progenitor cells. Journal of Cellular and Molecular Medicine.
2011;
15
(9)
:
1896-913
.
View Article PubMed Google Scholar -
Skiles
M.L.,
Marzan
A.J.,
Brown
K.S.,
Shamonki
J.M.,
Comparison of umbilical cord tissue-derived mesenchymal stromal cells isolated from cryopreserved material and extracted by explantation and digestion methods utilizing a split manufacturing model. Cytotherapy.
2020;
22
(10)
:
581-91
.
View Article PubMed Google Scholar -
Tsai
M.S.,
Lee
J.L.,
Chang
Y.J.,
Hwang
S.M.,
Isolation of human multipotent mesenchymal stem cells from second-trimester amniotic fluid using a novel two-stage culture protocol. Human Reproduction (Oxford, England).
2004;
19
(6)
:
1450-6
.
View Article PubMed Google Scholar -
Foty
R.,
A simple hanging drop cell culture protocol for generation of 3D spheroids. Journal of visualized experiments.
2011;
2011
(51)
:
2720
.
View Article PubMed Google Scholar -
Kim
T.H.,
Choi
J.H.,
Jun
Y.,
Lim
S.M.,
Park
S.,
Paek
J.Y.,
3D-cultured human placenta-derived mesenchymal stem cell spheroids enhance ovary function by inducing folliculogenesis. Scientific Reports.
2018;
8
(1)
:
15313
.
View Article PubMed Google Scholar -
Petrenko
Y.,
Syková
E.,
Kubinová
Š.,
The therapeutic potential of three-dimensional multipotent mesenchymal stromal cell spheroids. Stem Cell Research & Therapy.
2017;
8
(1)
:
94
.
View Article PubMed Google Scholar -
Lee
J.H.,
Han
Y.S.,
Lee
S.H.,
Long-Duration Three-Dimensional Spheroid Culture Promotes Angiogenic Activities of Adipose-Derived Mesenchymal Stem Cells. Biomolecules & Therapeutics.
2016;
24
(3)
:
260-7
.
View Article PubMed Google Scholar -
Bartosh
T.J.,
Ylöstalo
J.H.,
Mohammadipoor
A.,
Bazhanov
N.,
Coble
K.,
Claypool
K.,
Aggregation of human mesenchymal stromal cells (MSCs) into 3D spheroids enhances their antiinflammatory properties. Proceedings of the National Academy of Sciences of the United States of America.
2010;
107
(31)
:
13724-9
.
View Article PubMed Google Scholar -
Barkholt
L.,
Flory
E.,
Jekerle
V.,
Lucas-Samuel
S.,
Ahnert
P.,
Bisset
L.,
Risk of tumorigenicity in mesenchymal stromal cell-based therapies\textemdashbridging scientific observations and regulatory viewpoints. Cytotherapy.
2013;
15
(7)
:
753-9
.
View Article PubMed Google Scholar -
Blum
B.,
Benvenisty
N.,
The tumorigenicity of human embryonic stem cells. Advances in Cancer Research.
2008;
100
:
133-58
.
View Article PubMed Google Scholar -
Greco
S.J.,
Liu
K.,
Rameshwar
P.,
Functional similarities among genes regulated by OCT4 in human mesenchymal and embryonic stem cells. Stem Cells (Dayton, Ohio).
2007;
25
(12)
:
3143-54
.
View Article PubMed Google Scholar -
Cananzi
M.,
De Coppi
P.,
CD117(+) amniotic fluid stem cells: state of the art and future perspectives. Organogenesis.
2012;
8
(3)
:
77-88
.
View Article PubMed Google Scholar -
Li
C.,
Zhou
J.,
Shi
G.,
Ma
Y.,
Yang
Y.,
Gu
J.,
Pluripotency can be rapidly and efficiently induced in human amniotic fluid-derived cells. Human Molecular Genetics.
2009;
18
(22)
:
4340-9
.
View Article PubMed Google Scholar -
Moschidou
D.,
Mukherjee
S.,
Blundell
M.P.,
Drews
K.,
Jones
G.N.,
Abdulrazzak
H.,
Valproic acid confers functional pluripotency to human amniotic fluid stem cells in a transgene-free approach. Molecular Therapy.
2012;
20
(10)
:
1953-67
.
View Article PubMed Google Scholar -
Ditadi
A.,
de Coppi
P.,
Picone
O.,
Gautreau
L.,
Smati
R.,
Six
E.,
Human and murine amniotic fluid c-Kit+Lin- cells display hematopoietic activity. Blood.
2009;
113
(17)
:
3953-60
.
View Article PubMed Google Scholar -
Chiavegato
A.,
Bollini
S.,
Pozzobon
M.,
Callegari
A.,
Gasparotto
L.,
Taiani
J.,
Human amniotic fluid-derived stem cells are rejected after transplantation in the myocardium of normal, ischemic, immuno-suppressed or immuno-deficient rat. Journal of Molecular and Cellular Cardiology.
2007;
42
(4)
:
746-59
.
View Article PubMed Google Scholar -
Zhang
S.,
Geng
H.,
Xie
H.,
Wu
Q.,
Ma
X.,
Zhou
J.,
The heterogeneity of cell subtypes from a primary culture of human amniotic fluid. Cellular & Molecular Biology Letters.
2010;
15
(3)
:
424-39
.
View Article PubMed Google Scholar -
Markmee
R.,
Aungsuchawan
S.,
Narakornsak
S.,
Tancharoen
W.,
Bumrungkit
K.,
Pangchaidee
N.,
Differentiation of mesenchymal stem cells from human amniotic fluid to cardiomyocyte-like cells. Molecular Medicine Reports.
2017;
16
(5)
:
6068-76
.
View Article PubMed Google Scholar -
Sessarego
N.,
Parodi
A.,
Podestà
M.,
Benvenuto
F.,
Mogni
M.,
Raviolo
V.,
Multipotent mesenchymal stromal cells from amniotic fluid: solid perspectives for clinical application. Haematologica.
2008;
93
(3)
:
339-46
.
View Article PubMed Google Scholar -
Tsai
M.S.,
Hwang
S.M.,
Chen
K.D.,
Lee
Y.S.,
Hsu
L.W.,
Chang
Y.J.,
Functional network analysis of the transcriptomes of mesenchymal stem cells derived from amniotic fluid, amniotic membrane, cord blood, and bone marrow. Stem Cells (Dayton, Ohio).
2007;
25
(10)
:
2511-23
.
View Article PubMed Google Scholar -
Tsai
M.S.,
Hwang
S.M.,
Tsai
Y.L.,
Cheng
F.C.,
Lee
J.L.,
Chang
Y.J.,
Clonal amniotic fluid-derived stem cells express characteristics of both mesenchymal and neural stem cells. Biology of Reproduction.
2006;
74
(3)
:
545-51
.
View Article PubMed Google Scholar
Comments
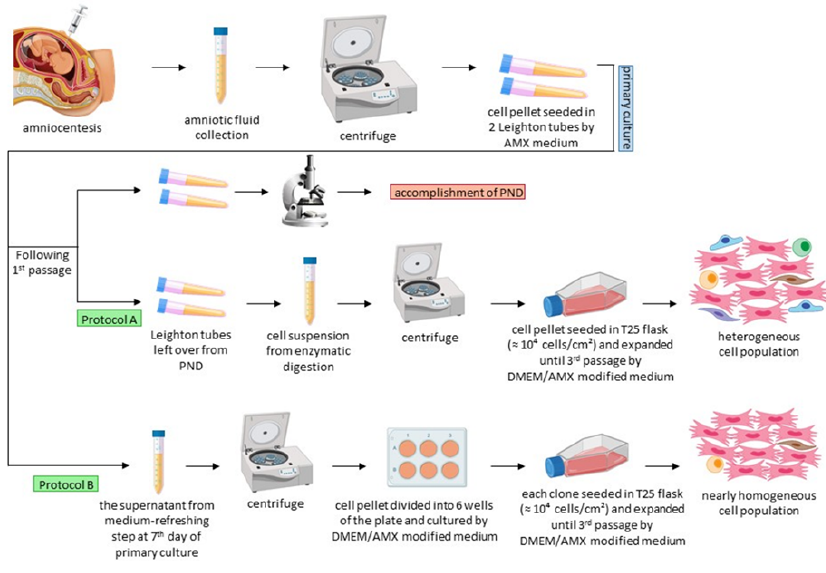
Article Details
Volume & Issue : Vol 11 No 8 (2024)
Page No.: 6683-6697
Published on: 2024-08-31
Citations
Copyrights & License

This work is licensed under a Creative Commons Attribution 4.0 International License.
Search Panel
Pubmed
Google Scholar
Pubmed
Google Scholar
Pubmed
Google Scholar
Pubmed
Google Scholar
Pubmed
Google Scholar
Pubmed
Google Scholar
Pubmed
Search for this article in:
Google Scholar
Researchgate
- HTML viewed - 1438 times
- PDF downloaded - 571 times
- XML downloaded - 43 times