Abstract
Introduction: Traditional medicine treatments (TMT) are widely recognized as effective complementary therapies in cancer care, including hepatocellular carcinoma (HCC). Tieu u hoan (TUH), an herbal formulation derived from TMT principles and clinical expertise, has shown promise in preclinical and clinical studies as a supportive therapy for HCC. This study investigates the immunomodulatory effects of TUH in immunodeficient nude mice engrafted with human Hep3B liver cancer cells.
Methods: Immune responses were evaluated in Hep3B tumor-bearing nude mice treated with TUH. Parameters included relative spleen weight, splenic histopathology (lymphoid follicle proliferation in white pulp), proportions of NK cells, dendritic cells (DCs), and macrophages in the spleen, plasma concentrations of IL-2 and TNF-α, and leukocyte/lymphocyte counts in peripheral blood.
Results: Administration of TUH (3.53 g/kg) significantly increased relative spleen weight (p < 0.05), enhanced lymphoid follicle proliferation in splenic white pulp, and elevated proportions of NK cells, DCs, and macrophages (p < 0.05). TUH also raised plasma TNF-α and IL-2 levels (p < 0.05) and increased peripheral leukocyte and lymphocyte counts (p < 0.05), suggesting potentiation of both innate and adaptive immunity.
Conclusion: TUH demonstrates significant immunoenhancing effects in a murine HCC model, providing a scientific basis for its use as a complementary therapy in HCC management. These findings underscore its potential to augment immune responses during conventional cancer treatment.
Introduction
Hepatocellular carcinoma (HCC) is a common malignancy and a leading cause of cancer-related mortality globally1. Vietnam ranks among countries with the highest HCC incidence, primarily attributed to the high prevalence of chronic hepatitis B and C virus infections2, 3, 4. Current standard therapies for HCC include surgical resection, liver transplantation, transarterial chemoembolization (TACE), radiofrequency ablation (RFA), chemotherapy, and molecular-targeted agents1, 5, 6, 7. While surgical resection and transplantation are first-line options for early-stage HCC6, 8, 9 intermediate-stage patients often receive locoregional therapies such as microwave ablation (MWA), a thermal ablation technique analogous to RFA that induces tumor necrosis via hyperthermia6, 10. Despite these advances, post-treatment recurrence remains a major clinical challenge. Repeated interventions risk further hepatic functional decline, potentially culminating in liver failure and mortality11. Consequently, strategies to prevent HCC recurrence are critical for improving long-term survival.
Traditional Medicine Therapy (TMT), particularly traditional herbal medicines (THMs), has been widely integrated into supportive cancer care, including adjunctive HCC management12, 13, 14. Preclinical studies have identified several THMs with potential to inhibit HCC progression and carcinogenesis15, 16, 17. Emerging evidence suggests that combining modern therapies with TMT may synergistically enhance immune function, reduce recurrence and metastasis risk, and alleviate chemotherapy- or radiation-induced toxicities, thereby improving clinical outcomes18, 19.
Tieu U Hoan (TUH), a multi-herbal formulation comprising 12 clinically utilized botanicals, is rooted in traditional medicinal principles. It is theorized to exert detoxifying, anti-tumor, and circulation-enhancing effects while supporting splenic and renal function. While modern research has characterized immunomodulatory properties of individual TUH components, the formulation’s holistic effects remain unexplored. This study investigates TUH’s immune-enhancing potential in a Hep-3B human HCC xenograft model using nude mice, aiming to provide preclinical evidence for its role in integrative liver cancer therapy.
Methods
Materials
TUH is a soft tablet weighing 8.5 g, containing 4.4 g of active ingredients and 4.1 g of excipients (honey). It is prepared at the Center for Research and Application of Traditional Medicine Production under the Military Traditional Medicine Institute, meeting basic quality standards. The ingredients of TUH include: Prunus persica, Carthamus tinctorius, Paeonia lactiflora, Rheum palmatum, Prunus armeniaca, Glycyrrhiza uralensis, Achyranthes bidentata, Whitmania pigra, Rehmannia glutinosa, Hedyotis diffusa, Scutellaria barbata, and Astragalus propinquus.
Experimental Drug: Based on the traditional dosage of 12 medicinal ingredients and the evaluation of active ingredient content in the pills, the recommended human dose of Tieu U Hoan is four pills per person per day, equivalent to 4 × 8,500 mg = 34,000 mg per person per day. For an average 60 kg adult, this corresponds to 567 mg/kg/day.
The conversion factor for human doses to nude mice is 6.220. The equivalent dose for nude mice is calculated as follows: ADE (mg/kg) = Human dose (mg/kg) × Km ratio20. ADE = 0.57 × 6.2 = 3.53 g/kg. The experimental groups were organized as follows: Group 2 was treated with the clinical dose of 3.53 g/kg body weight, and Group 3 was treated with three times the clinical dose, corresponding to 10.59 g/kg body weight.
Animals
In this study, twenty-four immunodeficient BALB/c (nude) mice, aged 6–8 weeks with an average weight of 18–30 g, regardless of sex, were provided by Life Science Development Company Limited and imported from Taiwan. The mice were transplanted with Hep-3B hepatocellular carcinoma cells and housed in a positive-pressure laboratory at the Experimental Animal Research Center, Vietnam Military Medical University. The room temperature was maintained at 26 ± 0.5°C, with humidity at 55 ± 5%. The lighting system was automatically controlled, with lights on from 07:00 to 19:00. Food (Zeigler, USA) and sterilized water were provided. Each cage was housed on an individually ventilated rack with a filter to ensure pathogen isolation.
Methods
Firstly, HCC cell (Hep-3B) culture and proliferation were performed. The cell lines were cultured in 10-cm dishes containing RPMI-1640 medium supplemented with 10% fetal bovine serum (FBS) and 1% penicillin-streptomycin. The cells adhered uniformly to the surface of the culture dishes. The culture medium was replaced every three days until cell coverage exceeded 80% of the dish area. Afterward, the cells were detached using 0.04% Trypsin-EDTA and transferred to a new culture dish. This cycle was repeated until a sufficient number of cells were obtained for experiments. The cancer cells were preserved in complete culture medium supplemented with 5% DMSO and stored in a liquid nitrogen tank. Finally, the cells were collected by centrifugation after Trypsin-EDTA treatment and resuspended evenly in fresh RPMI-1640 medium. Cell density was determined using a Neubauer counting chamber and a microscope.
Secondly, inoculating cancer cells into mice: The cancer cells were injected into the subcutaneous thigh region of each mouse at a concentration of 2 × 10⁶ cells/mouse (1 × 10⁶ cells per thigh) under sterile conditions. Tumor formation was evaluated by monitoring growth at the injection site twice a week through visual observation, palpation, and precise measurements using an NSK (Japan) caliper. Tumor dimensions were recorded twice weekly along two axes: length (D) and width (R). Tumor volume (V) was calculated using the following formula: V = D × R² × 0.5, where V is the tumor volume, D is the tumor length, and R is the tumor width21. When transplanting human liver cancer cells from the Hep-3B line, the tumor incidence rate reached 87.5% and was observed 7 days post-transplantation. No tumor regression was observed (0%). The average tumor volume measured 35 days post-transplantation was 774.8 mm³. No nude mice died due to procedural complications. Throughout the experiment, the pathological features of the tumors were similar to those observed in human tumors.
After implanting Hep-3B tumor cells, 24 mice were randomly divided into three treatment groups (8 mice per group) and administered different doses orally: Group 1 (Control group): Distilled water at 0.1 mL per 10 g of body weight for 30 days; Group 2 (Dose 1): TUH at 3.53 g/kg/day (equivalent to the clinical dose) for 30 days; Group 3 (Dose 2): TUH at 10.59 g/kg/day (three times the clinical dose) for 30 days. The TUH was dissolved in water and administered orally via gavage using a blunt-tipped needle. After the 30-day treatment period, blood and spleen samples were collected from the mice for immune stimulation assessment.
Evaluation criteria, Location and duration of the research
To evaluate the immunomodulatory effects of TUH preparation, various parameters were evaluated, including relative spleen weight, spleen histopathology, the proportion of NK cells, DC cells, and macrophages in the spleen, as well as plasma IL-2 and TNF-α concentrations and peripheral blood cell counts.
To evaluate the immunomodulatory effects of TUH, the following parameters were assessed: Relative spleen weight; spleen histopathology; proportions and immunophenotypes of NK cells, DCs, and macrophages in the spleen; plasma concentrations of IL-2 and TNF-α; peripheral blood cell counts.
The spleen was examined histopathologically at the Department of Pathology, Military Hospital 103, Vietnam Military Medical University. Quantification of IL-2, TNF-α, and peripheral blood cells was conducted at the Laboratory of Applied Molecular Biology, Institute of Microbiology and Biotechnology, Vietnam National University, Hanoi. IL-2 and TNF-α were quantified using kits provided by Invitrogen Corporation, a part of Thermo Fisher Scientific, USA. The proportions of NK cells, DCs, and macrophages in the spleens of nude mice were determined at the Department of Pathophysiology, Vietnam Military Medical University. Hematological tests were conducted at the Department of Paraclinical Pathology, Le Huu Trac National Burn Hospital, Vietnam Military Medical University. The study was conducted from March to August 2022.
Isolation and determination of Immune cell proportions from mouse spleen
The mice were surgically dissected to collect their spleens, which were thoroughly washed with PBS solution. Forceps were gently used to compress the tissue to release the inner cells. The isolated cells were incubated with red blood cell lysis buffer for 6–8 minutes. The cell populations were analyzed using a FACS Canto II flow cytometer (BD Biosciences) to determine the proportions of different cell types. The cells were classified based on size (FSC) and complexity (SSC). Subsequently, they were further categorized by fluorescence intensity (FITC and PE). The proportions of cells were analyzed using BD Canto Diva software22.
Trypan Blue Staining
Cell viability was evaluated using a Trypan Blue staining solution. Cell Sample Collection: Lyse red blood cells (repeat twice) and collect single cells. Dilute Trypan Blue at a 1:1 ratio with the cell sample. Incubate at room temperature for 3–5 minutes. Examine the sample under a microscope to assess the live/dead cell ratio. Removal of Trypan Blue: Centrifuge and wash the cells with PBS.
Specific Antibody Staining
Four tubes of 10⁶ cells obtained from each mouse by the procedure mentioned above were prepared. Each tube was stained separately with specific antibodies: anti-CD49b antibodies were labeled with FITC (specific for NK cell identification) 23, anti-CD11b antibodies were labeled with APC (specific for macrophage identification)24, anti-CD197 antibodies labeled with PerCP-Cy5-5 (specific for mDC identification)25, and anti-CD11c antibodies labeled with FITC (specific for DC identification)25.
Data analysis
The collected data were processed using appropriate medical statistical methods with IBM SPSS Statistics version 20.0 software. Results were presented as the mean (X̅) ± standard deviation (SD), and statistical significance was assessed using the Wilcoxon signed-rank test with a significance level of p < 0.05.
Results
Relative spleen weight and spleen histopathology images
To evaluate immune stimulation, spleen samples were collected from both control and treatment groups at the end of the experiment. Relative spleen weight (g/10 g body weight) was calculated as spleen weight (g) divided by mouse body weight (g), multiplied by 10. Among the treatment groups, mice receiving Dose 1 (3.53 g/kg TUH) exhibited the highest relative spleen weight (0.12 g/10 g), followed by the Dose 2 group (10.59 g/kg TUH) with 0.11 g/10 g. In contrast, the control group had the lowest relative spleen weight (0.08 g/10 g). Both TUH-treated groups showed an increase in relative spleen weight compared to the control group. However, a statistically significant difference (p < 0.05) was observed only in the Dose 1 group. No significant difference was detected between the Dose 2 and control groups. These findings suggest that the lower dose of TUH may exert a more pronounced immunostimulatory effect on spleen development (Figure 1).
The control group displayed a normal histological architecture with well-defined white pulp areas. In contrast, spleens from TUH-treated mice exhibited prominent lymphoid follicle proliferation within the white pulp, indicating increased lymphoid activity. These histological changes suggest enhanced immune activation in the treatment groups (Figure 2).
Immune phenotype and proportion of NK cells, DC cells, and macrophages in mouse spleen
Innate immune cells, including macrophages, dendritic cells (DCs), and natural killer (NK) cells, play a pivotal role in antitumor immunity by directly inducing cytotoxicity or inhibiting tumor growth without prior sensitization26. In this study, BD Canto Diva flow cytometry (FCM) was employed to characterize the immunophenotype and quantify the proportions of these cell populations in mouse spleens. Fluorescently labeled antibodies were used to distinguish immune cell subsets: FITC-conjugated anti-CD49b antibodies for NK cells and APC-conjugated anti-CD11b antibodies for macrophages.
Representative flow cytometry profiles from 24 spleen samples across the three study groups are shown in Figure 3 and Figure 4. Differences in immune cell distribution were observed among the groups, highlighting the impact of TUH administration on splenic immune cell composition.
Specifically, macrophage proportions were highest in the Dose 1 group (4.58%), followed by Dose 2 (3.60%) and the control group (3.28%), indicating an increase in macrophage representation in TUH-treated mice. Dendritic cell proportions exhibited a similar trend, with 18.67% in Dose 1, 14.32% in Dose 2, and 8.32% in the control group. NK cell frequencies were also elevated in the treated groups, reaching 2.96% in Dose 1, 1.89% in Dose 2, and 1.74% in the control group. Although increases in all three immune cell types were observed in both treatment groups, statistically significant differences (p < 0.05) compared to the control group were detected only in the Dose 1 group (3.53 g/kg TUH). Notably, the NK cell proportion in the Dose 1 group was significantly higher than in the Dose 2 group, suggesting a dose-dependent immunomodulatory effect of TUH on NK cell expansion (Figure 5). These findings suggest that TUH, particularly at the lower dose, may more effectively stimulate the innate immune system by enhancing macrophage, DC, and NK cell populations in the spleen.
Peripheral blood cell counts
White blood cell (WBC) counts were higher in the TUH-treated groups compared to the control group, indicating a potential stimulatory effect on systemic immunity. Specifically, the WBC count in the Dose 1 group (3.53 g/kg TUH) was 10.35 × 10⁹/L, markedly higher than that observed in the Dose 2 group (10.49 g/kg TUH), which was 4.83 × 10⁹/L, and the control group, which showed the lowest count at 3.91 × 10⁹/L. A statistically significant difference (p < 0.05) was observed between the Dose 1 and control groups. Furthermore, the WBC count in the Dose 1 group was significantly higher than in the Dose 2 group (p < 0.05), suggesting that the lower dose of TUH may be more effective in enhancing leukocyte production or mobilization. No significant differences were observed in red blood cell (RBC) or platelet counts across the three groups (Figure 6).
Lymphocyte counts were highest in mice treated with Dose 1 (3.53 g/kg TUH), reaching 4.73 × 10⁹/L, followed by the Dose 2 group (1.49 × 10⁹/L), while the control group exhibited the lowest value (1.19 × 10⁹/L). A statistically significant increase (p < 0.05) was observed in the Dose 1 group compared to both the control and Dose 2 groups, indicating that the lower dose of TUH significantly enhanced lymphocyte proliferation or mobilization. Neutrophil (N) counts were also elevated in TUH-treated mice compared to controls; however, these differences did not reach statistical significance (p > 0.05). Similarly, monocyte counts were higher in the Dose 1 group than in the control group, but the difference was not statistically significant (p > 0.05). These results suggest that TUH, particularly at the lower dose, has a pronounced effect on lymphocyte populations, while its influence on neutrophils and monocytes appears less substantial under the conditions tested (Figure 7).
Evaluation of interleukin-2 and tumor necrosis factor-alpha concentrations
As you know, IL-2 and TNF-α are two cytokines that play an important role in the immune response against cancer cells (10). Serum interleukin-2 (IL-2) levels were elevated in TUH-treated mice compared to the control group (p < 0.05), indicating enhanced immune activation. Specifically, IL-2 levels reached 66.93 pg/mL in the Dose 1 group and 58.46 pg/mL in the Dose 2 group, while the control group showed a lower concentration of 53.99 pg/mL (Figure 8).
Tumor necrosis factor-alpha (TNF-α) concentrations were elevated in both TUH-treated groups compared to the control group. Specifically, TNF-α levels were 88.87 pg/mL in the Dose 1 group and 108.54 pg/mL in the Dose 2 group, whereas the control group exhibited a markedly lower level of 20.95 pg/mL. Despite the numerical increase in both treatment groups, a statistically significant difference (p < 0.05) was observed only in the Dose 1 group (3.53 g/kg TUH) compared to the control. These findings suggest that TUH may enhance TNF-α production, particularly at the lower dose, contributing to its potential immunostimulatory or antitumor effects (Figure 9).
Discussion
The spleen, which is composed of red pulp and white pulp, is the largest blood-filtering organ in the body. It plays vital immunological roles, including hematopoiesis and red blood cell clearance. Moreover, its unique structure is critical for filtering blood-borne pathogens and antigens26. In addition to filtering and storing blood, the spleen also serves as a site for antigen concentration, particularly when antigens enter the bloodstream. After entering the bloodstream, antigens are processed by macrophages, which initially fix them in the red pulp and subsequently transport them to the white pulp. In the white pulp, lymphoid follicles, which are rich in lymphocytes, stimulate their differentiation into plasma cells27. In this study, the relative spleen weight of mice treated with TUH was higher than that of the control group. However, statistically significant differences were observed only in the group treated with 3.53 g/kg TUH (p < 0.05). The results of spleen histopathology further illustrate increased lymphoid follicle proliferation in the white pulp of TUH-treated mice, providing evidence for the observed differences in spleen weight among the groups. In the treatment groups, areas of the white pulp with proliferative lymphoid follicles were observed, suggesting that immune responses against tumors may lead to an expansion of the T-cell zone.
NK cells play a critical role in innate immune responses and exhibit natural cytotoxicity against various targets, including cancer cells and virus-infected cells, without requiring antigen specificity. A reduction in NK cell counts correlates with disease progression26, 28, 29. Dendritic cells (DCs) are present in lymphoid organs, blood, lymph fluid, and some non-lymphoid tissues, and they function as antigen-presenting cells for T lymphocytes30, 31. After capturing antigens in tissues, DCs migrate to lymphoid organs, where they present these antigens to lymphocytes.
The results presented in Figure 3, Figure 4 and Figure 5 indicate that Dose 1-treated groups exhibit an increased number of NK cells and DC cells in the spleen compared to the control group. The rise in dendritic cell numbers in the treated groups suggests the presence of antigens, while the increase in NK cell numbers reflects the body’s enhanced immune response against cancer.
Macrophages also play a crucial role in anticancer immune responses by facilitating the activation and potent lysis of cancer cells. They express receptors that enable them to engage cancer cells opsonized by antibodies, and through enzymatic processes within lysosomes, macrophages effectively eliminate cancer cells via oxidative reactions and other chemical mechanisms32, 33, 34. Figure 3 and Figure 5 show that the number of macrophages in the spleens of Dose 1-treated mice is higher than in the control group, indicating a greater capacity for cancer cell elimination in the treated groups.
The results in Figure 6 indicate that mice treated with TUH showed no significant differences in red blood cell (RBC) and platelet counts compared to the control group. Thus, TUH does not affect RBC and platelet levels in mice with Hep-3B tumors. In contrast, the number of white blood cells (WBCs) in peripheral blood reflects both innate and adaptive immune responses. Regular monitoring of WBC counts is a crucial hematological parameter for cancer patients undergoing drug or radiation therapy28, 29. In this research, the group receiving Dose 1 showed a higher WBC count than the control group, and this difference was statistically significant (p < 0.05).
Various types of peripheral blood leukocytes play essential roles in antigen recognition and contribute significantly to immune responses. Monitoring changes in these cell populations allows for a partial evaluation of the body's immune status, as each leukocyte type has distinct functions. For example, neutrophils constitute a substantial proportion of peripheral blood leukocytes and primarily function in phagocytosis and innate immune responses. Their numbers often increase during acute infections, as they are mobilized to sites of infection to recognize, attack, and destroy invading bacteria and viruses26, 30, 31, 32. Lymphocytes are essential components of adaptive immune responses. Based on differences in morphology, function, and surface markers, they are commonly classified into two major types: T lymphocytes and B lymphocytes. B lymphocytes primarily mediate humoral immunity, whereas T lymphocytes are crucial for cell-mediated immune responses33, 34. In this study, the lymphocyte count increased in the group treated with TUH at Dose 1, indicating an immunostimulatory effect of TUH (Figure 7).
The immune system comprises immune organs, specialized immune cells, and cytokines, all of which significantly influence the function of antigen-activated cells. Among these cytokines, IL-2—secreted by activated Th lymphocytes—plays a central role. IL-2 stimulates specific T lymphocytes, including both TCD4 and TCD8 cells. Additionally, it promotes the development and differentiation of B lymphocytes and enhances the activity of natural killer (NK) cells as well as lymphokine-activated killer (LAK) cells. When antigens bind to T cell receptors (TCR), T cells release IL-2, which then interacts with IL-2 receptors (IL-2R) to stimulate T-cell growth, differentiation, and survival. Moreover, IL-2 is essential for T-cell development in the thymus, boosts overall immune responses, and can lead to increased TCD4 cell counts11. IL-2 has demonstrated high efficacy in treating a range of cancers, including lung cancer, leukemia, ovarian cancer, breast cancer, hematological malignancies, renal cell carcinoma, and malignant melanoma. Both preclinical and clinical studies consistently emphasize its ability to stimulate and enhance immune cell functions, leading to effective eradication of cancer cells35.
Tumor Necrosis Factor Alpha (TNF-α) is a multifunctional cytokine involved in apoptosis, inflammation, and immune responses, and it is primarily synthesized by macrophages. Other cell types—including natural killer (NK) cells, granulocytes, T and B lymphocytes, adipocytes, fibroblasts, and endothelial cells—also contribute to TNF-α production. Its synthesis and secretion are chiefly stimulated by antigenic encounters, and TNF-α mediates its effects on target cells through specific receptors. Currently, TNF-α is employed in the treatment of certain connective tissue cancers, such as melanoma, primarily by destroying tumor blood vessels35, 36. Figure 8 and Figure 9 indicate that the group receiving a TUH dose of 3.53 g/kg exhibited significantly higher concentrations of both IL-2 and TNF-α compared to the control group (p < 0.05). This statistically significant difference suggests that TUH stimulates the immune system by enhancing cytokine secretion.
Significant differences were observed between the two treatment groups. In mice treated with TUH at 3.53 g/kg, compared to the control group, there was a notable increase in relative spleen weight, enhanced lymphoid tissue proliferation within the white pulp, and elevated total leukocyte and lymphocyte counts. Additionally, serum concentrations of IL-2 and TNF-α were significantly higher, and flow cytometric analysis revealed significantly increased proportions of NK cells, dendritic cells (DCs), and macrophages in the spleen (p < 0.05). In contrast, the 10.59 g/kg TUH group exhibited significant changes only in IL-2 serum concentration and lymphoid tissue proliferation in the spleen. Furthermore, the 3.53 g/kg group demonstrated significantly higher levels of NK cells, total white blood cells, and lymphocytes compared to the 10.59 g/kg group (p < 0.05). These findings suggest that TUH at a dose of 3.53 g/kg elicits a more robust immunostimulatory response. This may be attributed to the possibility that higher doses of TUH induce excessive immune activation, potentially exceeding the host’s regulatory capacity and leading to immunosuppressive effects. Therefore, the 3.53 g/kg dose appears to be more effective and may represent a more suitable candidate for clinical application, as it achieves optimal immune activation at a lower dose.
In traditional medicine, the treatment of liver cancer is based on the principle of syndrome differentiation and treatment, which involves combining various groups of herbs with different therapeutic effects to create a comprehensive approach. Instead of merely focusing on tumor eradication, the herbal formula also emphasizes enhancing the immune system, improving liver function, and maintaining internal balance. This holistic strategy enables the body to build sufficient resistance to fight cancer cells more effectively and safely37, 38.
Tieu U Hoan is skillfully formulated by combining multiple groups of medicinal herbs to establish a comprehensive mechanism for treating liver cancer, including clearing heat and detoxifying (Hedyotis diffusa Willd, Scutellaria barbata), promoting blood circulation and resolving stasis (Prunus persica, Carthamus tinctorius, Achyranthes bidentata, Hirudo), purging accumulation and resolving masses (Rheum palmatum Baill), and nourishing Qi and blood (Astragalus propinquus, Rehmannia glutinosa, Paeonia lactiflora). This balanced combination of herbs enhances immune modulation against cancer through the following mechanisms:
• Activation and enhancement of immune cell functions. Herbs such as Hedyotis diffusa, Scutellaria barbata, Astragalus membranaceus, and Glycyrrhiza uralensis enhance the activity of natural killer (NK) cells, which effectively recognize and eliminate cancer cells. Scutellaria barbata and Astragalus membranaceus stimulate the function of cytotoxic T cells, increasing their cytotoxic activity against liver cancer cells. Additionally, Hedyotis diffusa, Astragalus membranaceus, and Glycyrrhiza uralensis activate macrophages, enhancing their phagocytic capacity and promoting the secretion of anticancer cytokines such as TNF-α, IL-2, and IL-6, which further aid in the destruction of cancer cells37, 39.
• Regulation of cytokines and immune factors. Astragalus membranaceus, Hedyotis diffusa, Scutellaria barbata, and Glycyrrhiza uralensis stimulate the production of cytokines such as IL-2, IL-6, and IFN-α, thereby enhancing the immune response against liver cancer cells. By promoting the activation of T cells and NK cells, these herbs strengthen the body’s anticancer immunity. Furthermore, Hedyotis diffusa and Scutellaria barbata inhibit pro-inflammatory cytokines such as IL-10, reducing immunosuppression and allowing the immune system to function more effectively in eliminating cancer cells40, 37.
• Induction of apoptosis and inhibition of cancer cell growth. Hedyotis diffusa and Scutellaria barbata induce apoptosis in liver cancer cells, thereby reducing tumor size and preventing cancer progression. By activating intrinsic apoptosis pathways, these herbs effectively halt the proliferation of cancer cells. Additionally, Hedyotis diffusa and Scutellaria barbata inhibit angiogenesis around the tumor, restricting the supply of nutrients and oxygen to cancer cells, which consequently slows tumor growth and metastasis40, 41.
Antioxidant effects and liver protection. Hedyotis diffusa, Scutellaria barbata, Astragalus membranaceus, and Rehmannia glutinosa are rich in flavonoids, phenolic compounds, and polysaccharides, which provide strong antioxidant effects. These bioactive compounds neutralize free radicals, thereby protecting liver cells from oxidative stress and damage. This antioxidant activity is crucial for maintaining cellular integrity and preventing the transformation of healthy cells into cancerous ones42.
Immunomodulation and homeostatic balance. Astragalus membranaceus and Glycyrrhiza uralensis regulate the immune system by balancing Th1/Th2 (T-helper cell type 1/T-helper cell type 2) responses, thereby enhancing anticancer immunity without causing immune overactivity. By maintaining this balance, they optimize the body’s immune response, allowing it to effectively target cancer cells while minimizing the risk of autoimmune reactions. Additionally, Astragalus membranaceus and Glycyrrhiza uralensis boost the body’s overall resistance and adaptability. They enhance stress resilience, helping the body cope with the physiological and psychological stress associated with cancer and its treatments, including chemotherapy and radiotherapy. This adaptive capability not only supports immune function but also improves the patient's overall well-being and recovery process37.
Synergistic immunomodulatory and anticancer effects. The herbal components work synergistically to enhance immune function and directly inhibit cancer cell growth, making them particularly effective in the treatment of liver cancer. This dual action is achieved through a balanced combination that not only stimulates the immune system but also targets cancer cells with cytotoxic effects.
Therefore, Tieu U Hoan, with its harmonious combination of herbs that clear heat and detoxify, promote blood circulation and resolve stasis, and nourish Qi and blood, not only enhances immune function but also inhibits cancer cell growth, protects the liver, and maintains homeostatic balance. This comprehensive mechanism effectively strengthens the body's natural resistance, supporting liver cancer treatment in a safe and efficacious manner. By integrating immune modulation, direct anticancer effects, liver protection, and overall balance, Tieu U Hoan provides a holistic and powerful approach to combating liver cancer.
Conclusions
This study evaluated the immunomodulatory effects of TUH in nude mice bearing human Hep-3B liver cancer. Administration of TUH at a dose of 3.53 g/kg body weight significantly enhanced multiple immunological parameters compared to controls. Specifically, TUH treatment led to a marked increase in relative spleen weight, enhanced proliferation of lymphoid follicles in the splenic white pulp, and elevated proportions of NK cells, dendritic cells, and macrophages. Furthermore, both peripheral blood leukocyte and lymphocyte counts, as well as serum levels of TNF-α and IL-2, were significantly higher in the TUH-treated group (p < 0.05). Collectively, these findings provide robust evidence supporting the immunomodulatory activity of TUH and underscore its potential for further investigation in cancer immunotherapy.
Abbreviations
ADE (Animal equivalent dose), DC cell (Dendritic Cells), DMSO (Dimethyl Sulfoxide), FBS (Fetal bovine serum), HCC (Hepatocellular carcinoma), IL-10 (Interleukin-10), IL-2 (Interleukin-2), IL-2R (Interleukin-2 receptors), IL-6 (Interleukin-6), LAK (Lymphokine-activated killer), NK cell (Natural killer cell), RBC (Red blood cell), RPMI (Roswell Park Memorial Institute), TCR (T cell receptors), Th2 (T-helper cell type 2), THM (Traditional herbal medicines), TMT (Traditional medicine treatments), TNF-α (Tumor necrosis factor-alpha), TUH (Tieu u hoan), Th1 (T-helper cell type 1), and WBCs (White blood cells)
Acknowledgments
None.
Author’s contributions
Hai Song Nguyen: Research Investigator, Data Collection, Data Analysis, Writing – Original Draft. Hung Truong Thanh Luu: Research Supervision, Writing – Review & Editing, Project Administration. All authors have read and approved the final version of the manuscript.
Funding
This study was supported by a research grant from the Department of Defense. The funding agency had no involvement in the study design, data collection, analysis, interpretation, or manuscript preparation.
Availability of data and materials
Data and materials used and/or analyzed during the current study are available from the corresponding author on reasonable request.
Ethics approval
Animals in the study were cared for in a standard laboratory, and the studies were conducted under the guidance of the Experimental Animal Research Center, Vietnam Military Medical University. This study is a Ministry of National Defense-level project. The project description was approved by the Military Institute of National Medicine on October 11, 2021. The council overseeing the study was established under Decision No. 447/QD-VYHCTQD, issued on October 19, 2021, by the Director of the Military Traditional Medicine Institute.
Consent for publication
Not applicable.
Competing interests
The authors declare that they have no competing interests.
References
-
Abdelhamed
W.,
El-Kassas
M.,
Hepatocellular carcinoma recurrence: predictors and management. Liver Research.
2023;
7
(4)
:
321-32
.
View Article PubMed Google Scholar -
Huy Do
S.,
Epidemiology of hepatitis B and C virus infections and liver cancer in Vietnam. Euroasian Journal of Hepato-Gastroenterology.
2015;
5
(1)
:
49-51
.
View Article PubMed Google Scholar -
Le
V.Q.,
Nguyen
V.H.,
Nguyen
V.H.,
Nguyen
T.L.,
Sudenga
S.L.,
Trinh
L.H.,
Epidemiological Characteristics of Advanced Hepatocellular Carcinoma in the Northern Region of Vietnam. Cancer Control.
2019;
26
(1)
:
1073274819862793
.
View Article PubMed Google Scholar -
Nguyen-Dinh
S.H.,
Do
A.,
Pham
T.N.,
Dao
D.Y.,
Nguy
T.N.,
Chen
M.S.,
High burden of hepatocellular carcinoma and viral hepatitis in Southern and Central Vietnam: experience of a large tertiary referral center, 2010 to 2016. World Journal of Hepatology.
2018;
10
(1)
:
116-23
.
View Article PubMed Google Scholar -
Maluccio
M.,
Covey
A.,
Recent progress in understanding, diagnosing, and treating hepatocellular carcinoma. CA: a Cancer Journal for Clinicians.
2012;
62
(6)
:
394-9
.
View Article PubMed Google Scholar -
Arizumi
T.,
Ueshima
K.,
Minami
T.,
Kono
M.,
Chishina
H.,
Takita
M.,
Effectiveness of Sorafenib in Patients with Transcatheter Arterial Chemoembolization (TACE) Refractory and Intermediate-Stage Hepatocellular Carcinoma. Liver Cancer.
2015;
4
(4)
:
253-62
.
View Article PubMed Google Scholar -
Cheng
A.L.,
Kang
Y.K.,
Chen
Z.,
Tsao
C.J.,
Qin
S.,
Kim
J.S.,
Efficacy and safety of sorafenib in patients in the Asia-Pacific region with advanced hepatocellular carcinoma: a phase III randomised, double-blind, placebo-controlled trial. The Lancet. Oncology.
2009;
10
(1)
:
25-34
.
View Article PubMed Google Scholar -
Llovet
J.M.,
Ricci
S.,
Mazzaferro
V.,
Hilgard
P.,
Gane
E.,
Blanc
J.F.,
Investigators Study Group
SHARP,
Sorafenib in advanced hepatocellular carcinoma. The New England Journal of Medicine.
2008;
359
(4)
:
378-90
.
View Article PubMed Google Scholar -
Llovet
J.M.,
Villanueva
A.,
Marrero
J.A.,
Schwartz
M.,
Meyer
T.,
Galle
P.R.,
Panel of Experts on Trial Design in HCC
AASLD,
Trial Design and Endpoints in Hepatocellular Carcinoma: AASLD Consensus Conference. Hepatology (Baltimore, Md.).
2021;
73
(S1)
:
158-91
.
View Article PubMed Google Scholar -
Yu
Z.,
Geng
J.,
Zhang
M.,
Zhou
Y.,
Fan
Q.,
Chen
J.,
Treatment of osteosarcoma with microwave thermal ablation to induce immunogenic cell death. Oncotarget.
2014;
5
(15)
:
6526-39
.
View Article PubMed Google Scholar -
Wendon
J.,
Cordoba
J.,
Dhawan
A.,
Larsen
F.S.,
Manns
M.,
Samuel
D.,
Governing Board representative
EASL,
EASL Clinical Practical Guidelines on the management of acute (fulminant) liver failure. Journal of Hepatology.
2017;
66
(5)
:
1047-81
.
View Article PubMed Google Scholar -
Wu
P.,
Dugoua
J.J.,
Eyawo
O.,
Mills
E.J.,
Traditional Chinese Medicines in the treatment of hepatocellular cancers: a systematic review and meta-analysis. Journal of Experimental & Clinical Cancer Research.
2009;
28
(1)
:
112
.
View Article PubMed Google Scholar -
Chen
Q.,
Shu
C.,
Laurence
A.D.,
Chen
Y.,
Peng
B.G.,
Zhen
Z.J.,
Effect of Huaier granule on recurrence after curative resection of HCC: a multicentre, randomised clinical trial. Gut.
2018;
67
(11)
:
2006-16
.
View Article PubMed Google Scholar -
Song
X.,
Li
Y.,
Zhang
H.,
Yang
Q.,
The anticancer effect of Huaier (Review). Oncology Reports.
2015;
34
(1)
:
12-21
.
View Article PubMed Google Scholar -
Ma
J.,
Chen
M.,
Dai
Y.,
Enhancing the efficacy of photodynamic therapy by a Chinese herbal medicine for hepatocellular carcinoma. Cancer biology & therapy.
2006;
5
(9)
:
1117-9
.
View Article Google Scholar -
Gao
J.,
Lu
W.F.,
Dai
Z.J.,
Lin
S.,
Zhao
Y.,
Li
S.,
Induction of apoptosis by total flavonoids from Scutellaria barbata D. Don in human hepatocarcinoma MHCC97-H cells via the mitochondrial pathway. Tumour Biology.
2014;
35
(3)
:
2549-59
.
View Article PubMed Google Scholar -
Chen
M.W.,
Chen
W.R.,
Zhang
J.M.,
Long
X.Y.,
Wang
Y.T.,
Lobelia chinensis: chemical constituents and anticancer activity perspective. Chinese Journal of Natural Medicines.
2014;
12
(2)
:
103-7
.
View Article PubMed Google Scholar -
Yuan
H.,
Ma
Q.,
Ye
L.,
Piao
G.,
The Traditional Medicine and Modern Medicine from Natural Products. Molecules (Basel, Switzerland).
2016;
21
(5)
:
559
.
View Article PubMed Google Scholar -
Wei
L.,
Wang
Z.,
Jing
N.,
Lu
Y.,
Yang
J.,
Xiao
H.,
Frontier progress of the combination of modern medicine and traditional Chinese medicine in the treatment of hepatocellular carcinoma. Chinese Medicine.
2022;
17
(1)
:
90
.
View Article PubMed Google Scholar -
Nair
A.B.,
Jacob
S.,
A simple practice guide for dose conversion between animals and human. Journal of Basic and Clinical Pharmacy.
2016;
7
(2)
:
27-31
.
View Article PubMed Google Scholar -
Teicher
B.A.,
Tumor models in cancer research: Springer Science & Business Media; 2010Springer Science & Business Media 2010.
Google Scholar -
Martin-Jaular
L.,
Ferrer
M.,
Calvo
M.,
Rosanas-Urgell
A.,
Kalko
S.,
Graewe
S.,
Strain-specific spleen remodelling in Plasmodium yoelii infections in Balb/c mice facilitates adherence and spleen macrophage-clearance escape. Cellular Microbiology.
2011;
13
(1)
:
109-22
.
View Article PubMed Google Scholar -
Pellicci
D.G.,
Hammond
K.J.,
Coquet
J.,
Kyparissoudis
K.,
Brooks
A.G.,
Kedzierska
K.,
DX5/CD49b-positive T cells are not synonymous with CD1d-dependent NKT cells. The Journal of Immunology : Official Journal of the American Association of Immunologists.
2005;
175
(7)
:
4416-25
.
View Article PubMed Google Scholar -
Lloyd
C.M.,
Phillips
A.R.,
Cooper
G.J.,
Dunbar
P.R.,
Three-colour fluorescence immunohistochemistry reveals the diversity of cells staining for macrophage markers in murine spleen and liver. Journal of Immunological Methods.
2008;
334
(1-2)
:
70-81
.
View Article PubMed Google Scholar -
Lu
L.,
Hsieh
M.,
Oriss
T.B.,
Morel
P.A.,
Starzl
T.E.,
Rao
A.S.,
Generation of DC from mouse spleen cell cultures in response to GM-CSF: immunophenotypic and functional analyses. Immunology.
1995;
84
(1)
:
127-34
.
PubMed Google Scholar -
Xue
D.,
Lu
S.,
Zhang
H.,
Zhang
L.,
Dai
Z.,
Kaufman
D.S.,
Induced pluripotent stem cell-derived engineered T cells, natural killer cells, macrophages, and dendritic cells in immunotherapy. Trends in Biotechnology.
2023;
41
(7)
:
907-22
.
View Article PubMed Google Scholar -
Nguyen
D.H.,
Nguyen
T.S.,
Le
T.H.,
Nguyen
Q.U.,
Bui
N. Le,
Chu
D.T.,
Evaluation of the safety and immune stimulatory effects of multi-strain Lab Mix product on laboratory animals. Heliyon.
2024;
10
(2)
:
e24691
.
View Article PubMed Google Scholar -
Moretta
L.,
Moretta
A.,
Unravelling natural killer cell function: triggering and inhibitory human NK receptors. The EMBO Journal.
2004;
23
(2)
:
255-9
.
View Article PubMed Google Scholar -
Vitale
M.,
Cantoni
C.,
Pietra
G.,
Mingari
M.C.,
Moretta
L.,
Effect of tumor cells and tumor microenvironment on NK-cell function. European Journal of Immunology.
2014;
44
(6)
:
1582-92
.
View Article PubMed Google Scholar -
de Winde
C.M.,
Munday
C.,
Acton
S.E.,
Molecular mechanisms of dendritic cell migration in immunity and cancer. Medical Microbiology and Immunology.
2020;
209
(4)
:
515-29
.
View Article PubMed Google Scholar -
Alcántara-Hernández
M.,
Leylek
R.,
Wagar
L.E.,
Engleman
E.G.,
Keler
T.,
Marinkovich
M.P.,
High-Dimensional Phenotypic Mapping of Human Dendritic Cells Reveals Interindividual Variation and Tissue Specialization. Immunity.
2017;
47
(6)
:
1037-1050.e6
.
View Article PubMed Google Scholar -
Naito
M.,
Macrophage differentiation and function in health and disease. Pathology International.
2008;
58
(3)
:
143-55
.
View Article PubMed Google Scholar -
Gordon
S.,
The macrophage: past, present and future. European journal of immunology.
2007;
37
(S1)
:
S9-17
.
View Article Google Scholar -
Bonnardel
J.,
Guilliams
M.,
Developmental control of macrophage function. Current Opinion in Immunology.
2018;
50
:
64-74
.
View Article PubMed Google Scholar -
Shankar
A.,
Wang
J.J.,
Rochtchina
E.,
Yu
M.C.,
Kefford
R.,
Mitchell
P.,
Association between circulating white blood cell count and cancer mortality: a population-based cohort study. Archives of Internal Medicine.
2006;
166
(2)
:
188-94
.
View Article PubMed Google Scholar -
Grimm
R.H.,
Neaton
J.D.,
Ludwig
W.,
Prognostic importance of the white blood cell count for coronary, cancer, and all-cause mortality. Journal of the American Medical Association.
1985;
254
(14)
:
1932-7
.
View Article PubMed Google Scholar -
Liao
X.,
Bu
Y.,
Jia
Q.,
Traditional Chinese medicine as supportive care for the management of liver cancer: Past, present, and future. Genes {&}amp; Diseases.
2019;
7
(3)
:
370-9
.
View Article PubMed Google Scholar -
Ling
C.Q.,
Fan
J.,
Lin
H.S.,
Shen
F.,
Xu
Z.Y.,
Lin
L.Z.,
Chinese Integrative Therapy of Primary Liver Cancer Working Group
Clinical practice guidelines for the treatment of primary liver cancer with integrative traditional Chinese and Western medicine. Journal of Integrative Medicine.
2018;
16
(4)
:
236-48
.
View Article PubMed Google Scholar -
Yadav
N.,
Deshmukh
R.,
Majumder
R.J.P.R.-M.C.M.,
A comprehensive review on the use of traditional Chinese medicine for cancer treatment. Pharmacological Research-Modern Chinese Medicine.
2024;
11
:
100423
.
View Article Google Scholar -
Agrawal
K.K.,
Murti
Y.J.,
A systematic review on anticancer potential of Traditional Chinese Medicine (Yang Zheng XiaoJi). Pharmacological Research-Modern Chinese Medicine.
2024;
13
:
100505
.
View Article Google Scholar -
Hou
C.,
Wen
X.,
Yan
S.,
Gu
X.,
Jiang
Y.,
Chen
F.,
Network-based pharmacology-based research on the effect and mechanism of the Hedyotis diffusa-Scutellaria Barbata pair in the treatment of hepatocellular carcinoma. Scientific Reports.
2024;
14
(1)
:
963
.
View Article PubMed Google Scholar -
Ho
W.S.,
Active phytochemicals from chinese herbal medicines: anti-cancer activities and mechanisms.CRC Press 2015.
View Article Google Scholar
Comments
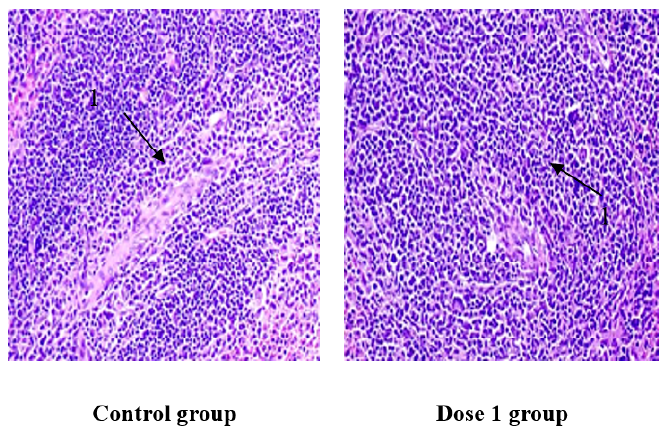
Article Details
Volume & Issue : Vol 12 No 4 (2025)
Page No.: 7335-7349
Published on: 2025-04-30
Citations
Copyrights & License

This work is licensed under a Creative Commons Attribution 4.0 International License.
Search Panel
- HTML viewed - 177 times
- PDF downloaded - 45 times
- XML downloaded - 17 times